Nuclear power plants produce around 20% of US electricity and the majority in France. Yet the industry is stagnant. Let's look at why.
Examining Cost
Operating cost matters for existing, depreciated nuclear power plants. Construction costs determine if investors will fund new power plants.
Operating Cost
Nuclear power plants have low fuel costs, meaning a large portion of their operating cost is fixed. Instead of buying fuel regularly like a coal or natural gas power plant, traditional civil nuclear plants refuel every 12-18 months. Nuclear fuel cycles create incentives similar to fixed costs.
Lazard estimates that a nuclear plant's operating costs vary between $28/MWh and $30/MWh.[1] Fuel is only 1/3 of that cost. A nuclear power plant has fixed operating costs 8x larger per kW than a natural gas combined cycle power plant and 2-3x a coal-fired power plant. Higher fixed costs come from a workforce that nuclear plants require to satisfy regulators, maintain higher safety standards, and manage the extra complexity nuclear technology brings. Operating costs would fall to ~$20/MWh if assuming a rate closer to coal power plants (which have similar power generating equipment). While existing plants likely suffer too much regulation, traditional nuclear power plant operation is more complex than a coal or gas plant. The plants would require more engineering talent and overhead even with zero regulation.
When I analyzed the largest US electricity market, PJM, for my solar post, I found that in 2019 and 2020, wholesale prices were only averaging around $25/MWh due to cheap natural gas. Even a completely paid-off nuclear plant can barely make ends meet in most US electricity markets. Existing plants in deregulated markets need their entire regulatory burden removed to stay open. There is a reason plants have been closing, or state legislatures are scrambling to work out subsidy deals.
The situation will deteriorate further. Daylight electricity prices in PJM averaged $29/MWh. Lazard estimates that new, unsubsidized utility-scale solar can compete at $30/MWh and as low as $25/MWh with the federal tax credit. New solar will lower daytime electricity prices, dropping average wholesale prices further. PJM is one of the worst markets for solar, and it still has almost 30 GW of solar applying for interconnection. Once solar is online, its total operating costs are under $5/MWh.
Traditional, depreciated nuclear power plants barely break even with a lighter regulatory burden. Expensive markets, like California ISO, are hostile to nuclear because opposition to energy is stiff.
Construction Cost
Traditional nuclear power plants are expensive to build. Historical costs range from <$1000/kW to infinite in the case of construction planning disasters like the attempted VC Summer plant expansion. Lazard estimates $8000-$12,000/kW based on plants under construction in the US, UK, and France.
Pecularities of Nuclear CAPEX Estimates
Dollars per kilowatt are the units for nuclear power plant construction costs. Numbers do not include the full scope of costs and are known as Overnight Construction Cost (OCC). OCC does not include interest payments during construction or decommissioning. Since governments often subsidize decommissioning and interest rates for nuclear power plants, OCC is better for comparison purposes.
Interest payments during construction can account for a large portion of upfront costs. The interest accounts for 12% of construction costs for a plant built in five years with a five percent interest rate. But if construction extends to 10 years with a 15% interest rate, the payments are more than the OCC.
Any OCC number is incomplete in understanding nuclear power economics.
Historical Costs
Nuclear power plant cost varies dramatically by country and period. Lovering, Yip, and Nordhaus compiled historical OCC data in their 2016 paper.[2]
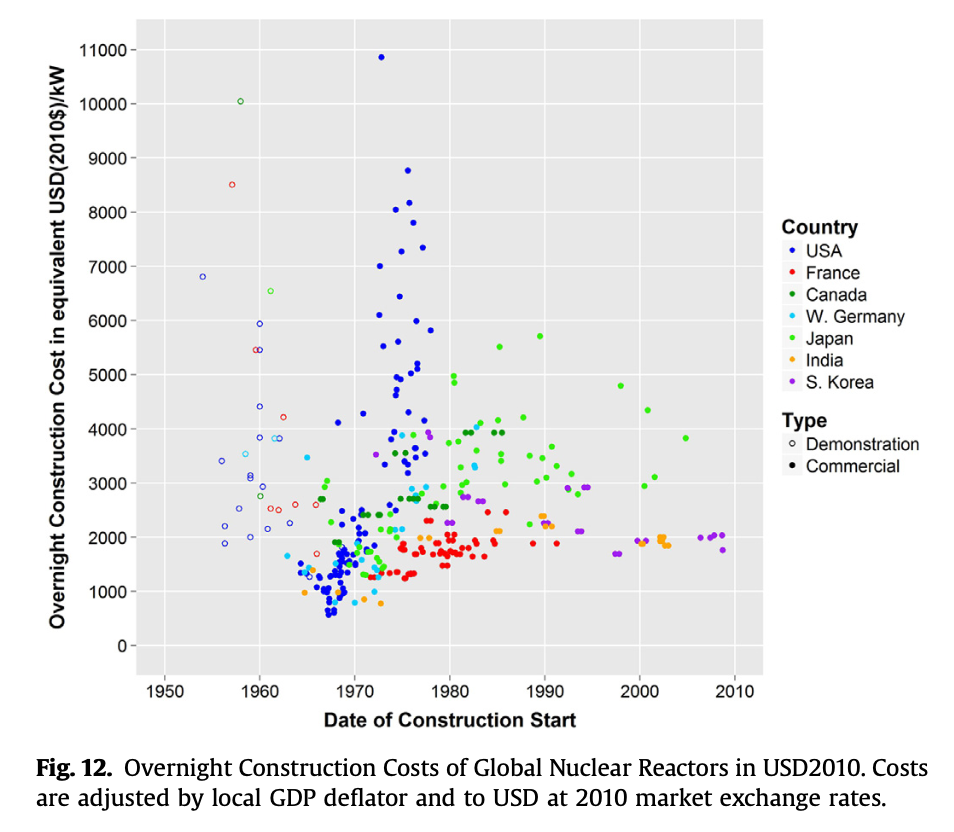
Lovering, Yip, Nordhaus
The first demonstration plants were expensive. Prices fell initially from scaling effects. Developers built many early nuclear plants in the US on underbid fix-priced contracts to establish their business. The pricing was not sustainable because of the underbidding, and these stripped-down designs were underwhelming in safety and reliability. The US and other markets saw cost increases as designers added safety and reliability features. US costs skyrocketed after Three Mile Island, while most other countries saw milder increases. A few countries like South Korea have embarked on building programs that utilize best practices to return near $2000/kW.
With experienced construction teams and engaged regulators, overnight construction costs for new plants can come in under $2000/kWh. Inexperienced construction teams and out-of-touch regulators can inflate the price by five times (or infinitely). The US, France, India, West Germany, and South Korea have seen periods of $2000/kW construction costs. Only India and South Korea have managed this feat recently.
Today's Competitive Landscape
Even when costs are low, construction times are long. Pre-Three Mile Island US nuclear plants had median construction times over five years. France saw similar timelines for its buildout. New plants are in countries where government-subsidized low-interest loans improve economics.
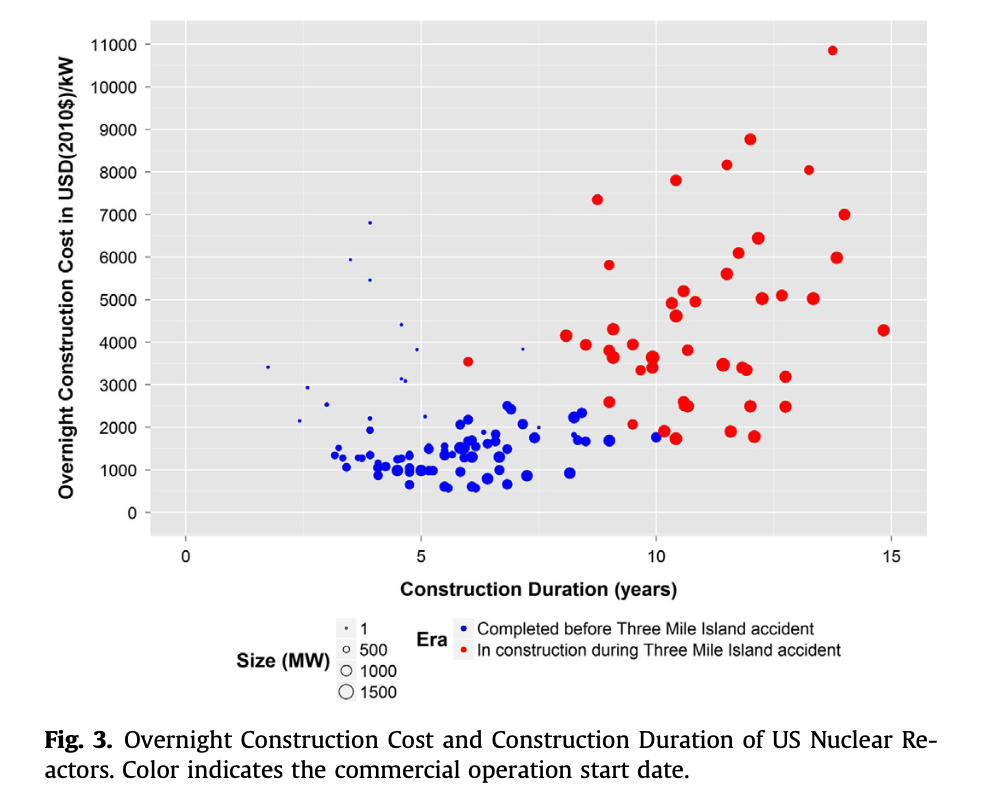
Lovering, Yip, Nordhaus
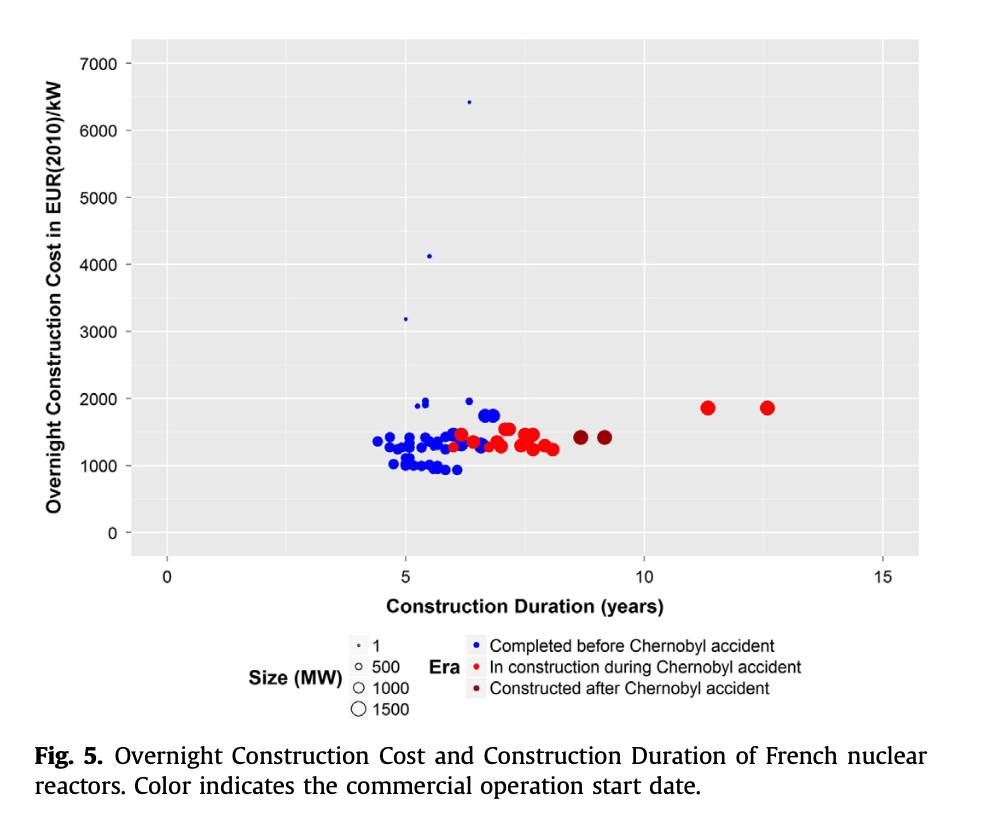
Lovering, Yip, Nordhaus
Technologies with low construction intensity like solar, wind, and natural gas turbines dominate the new power plant market. A $2000/kW plant built in under six years would equate to $34/MWh in capital costs. A low regulatory burden nuclear power plant would require over $54/MWh to break even. With interest-free loans, the total still comes to $46/MWh. Even if you could build a reactor with low-interest loans, government-subsidized decommissioning, plus 1960s regulation and labor costs, you'd still go bankrupt in competitive markets.
Learning Curves to the Rescue?
Manufacturing learning curves have led to dramatic falls in battery, solar panel, and wind turbine prices. Can nuclear see the same?
The Heat Engine Problem
Heat engines convert heat to work, like turning a generator. Heat engines can never be 100% efficient. The greater the temperature differential between the hot fluid and the cold fluid, the better efficiency is.
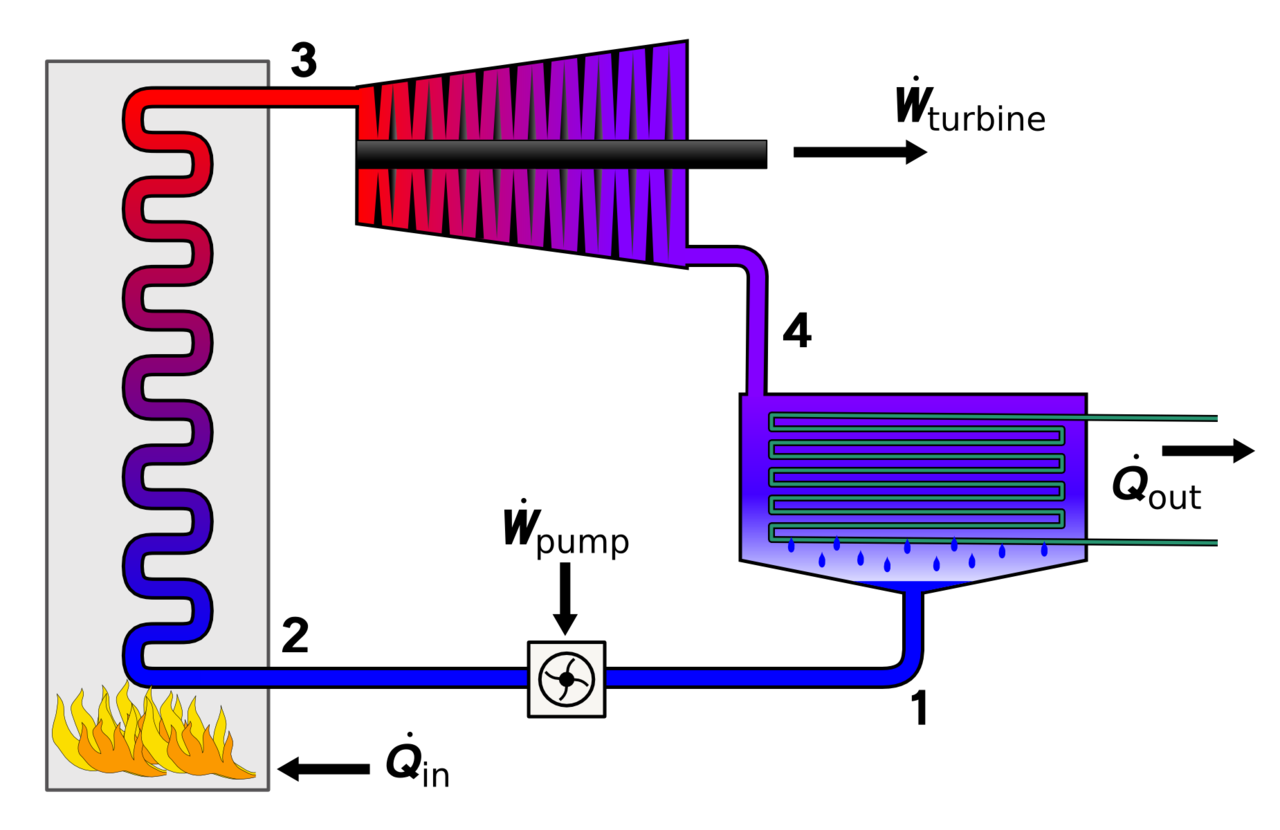
Physical layout of the Rankine cycle 1. Pump, 2. Boiler, 3. Turbine, 4. Condenser. Q = Heat and W = Work. Source: Andrew Ainsworth
Traditional thermal power plants, including nuclear and coal, heat water to make steam. The steam powers a turbine that turns a generator, producing electricity. The equipment and operation of the steam cycle are inherently expensive compared to gas turbines or solid-state technologies like solar panels. Hence, the heat engine problem.
Light Water Reactors
Most nuclear power plants are in a design family known as Light Water Reactors (LWR). The designs use water to cool the reactor and as a working fluid to drive a turbine. The reactor portion of the plant is only ~25% of construction costs.[3]
The rest of the facility is typical power plant components like heat exchangers, pumps, cooling towers, turbines, generators, etc. Nuclear plants have requirements like cobalt-free components, but other processing industries have similar limitations. These technologies are already far down a learning curve measured in hundreds of years, limiting potential cost declines.
LWRs have low efficiency, typically between 30% and 35%. A modern combined-cycle natural gas power plant is over 60% efficient. Low efficiency means more equipment and workers to produce the same amount of electricity. Improving LWR efficiency requires higher steam temperatures and pressures.
For the two main LWR designs, pressurized water reactors (PWR) and boiling water reactors (BWR), basic materials technology and economics limit the pressure and temperature of steam the plant can make. While fossil power plants often use superheated steam that allows more efficient turbine operation, PWRs and BWRs typically use saturated or slightly superheated steam.
The steam is saturated by definition in BWRs because it boils in the reactor vessel. BWRs run steam directly from the reactor to the turbine, meaning the turbine, condenser, and feedwater systems become irradiated. PWRs have a pressurized water loop cooling the reactor that transfers heat by boiling clean water in a heat exchanger known as a steam generator. Only the loop water becomes radioactive. PWRs can superheat steam, but the economics of the steam generator limit it. Boiling water has ten times the heat transfer of superheated steam, requiring a dramatically larger heat exchanger to superheat. In a fossil plant boiler, the firing temperature of the coal, gas, or oil might be hundreds of degrees higher than the steam. Because PWRs use pressure to prevent reactor loop water from boiling, the temperature differential is much tighter. Heat transfer increases with temperature differential, so the superheated steam heat transfer penalty for fossil-fired plants is lower.
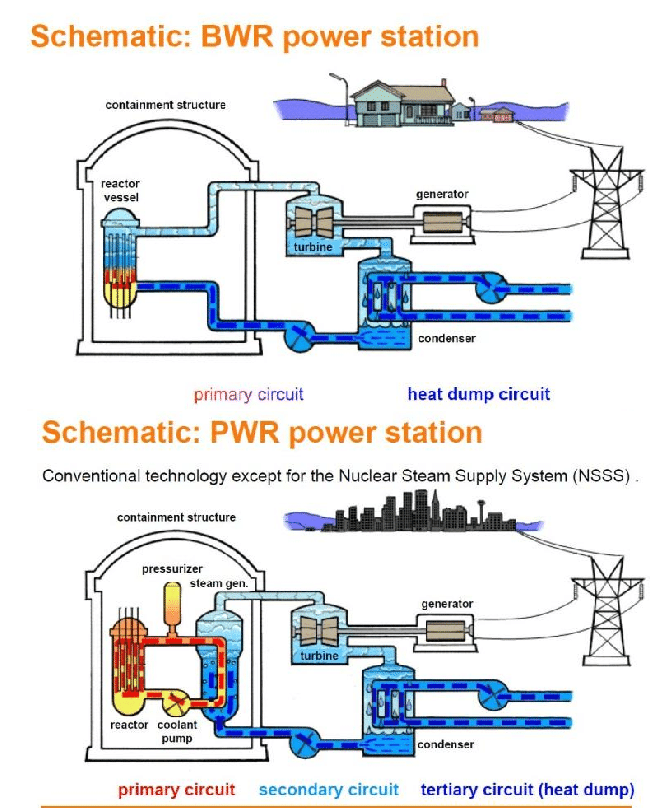
Source
If better efficiency isn't realistic, what is available? Longer fuel cycles can help, but the government places legal limits on the enrichment of LWR fuel for safety and proliferation reasons. Some new designs use natural circulation within the reactor loop water, providing some savings. Data from the Lovering paper suggests that standardizing a plant design, building plants in pairs, and having a consistent regulatory regime can lower costs, like in S. Korea.
In a post for his Construction Physics blog, Brian Potter did a deep dive into learning curves in the construction industry.[4] Learning curves exist, but they are reset by any design change, moving to a new site, or changing crews. The findings closely match what Lovering, Yip, and Nordhaus found drove cost reduction or escalation. It is unlikely there is a steep learning curve for LWRs below historical costs. Design and regulatory consistency paired with multiple copies at one site can mitigate cost inflation.
An anecdote from the massively overbudget Vogle Reactors 3 and 4 illuminates how inexperience interacts with regulations and safety requirements. In 1974, a candle started an insulation fire in the Brown's Ferry Nuclear Plant, burning a cable tray. [6] Operators prevented a meltdown, but independent safety systems went offline because their control wiring shared a cable tray. If one fire can disable redundant safety mechanisms then they aren't redundant. Cable tray separation of critical safety systems has been a core principle of nuclear safety since and came before Three Mile Island in 1979.
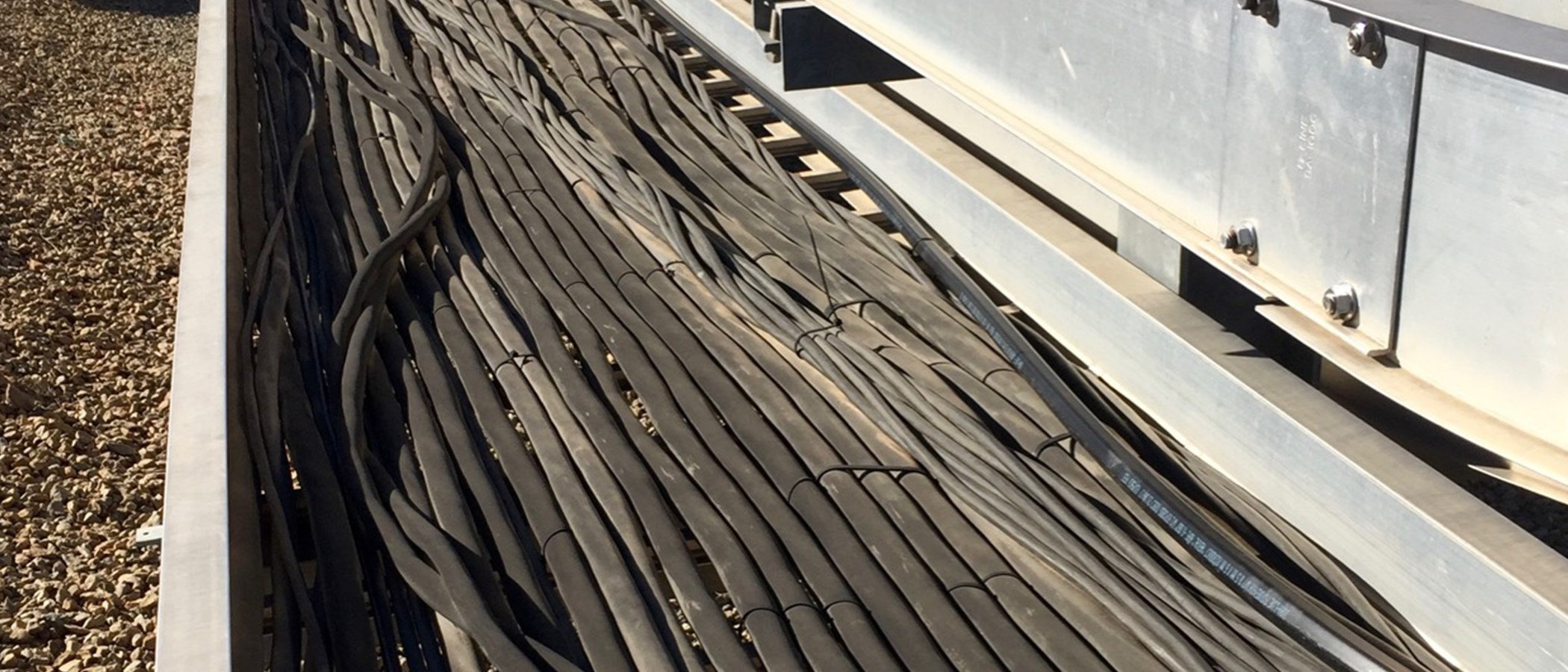
A Cable Tray Source: Duraline
In 2021, the Nuclear Regulatory Commission (NRC) dinged Southern Company for over 600 cable separation discrepancies. [5] Southern has to pay to fix it and pay interest on the loans through the delays. It is horrifically expensive since so many cables are in a tray. Is this regulation costly and lengthening construction timelines? Yes. Is this regulation based on the history of operating power plants safely? Yes. Would Southern repeat this mistake if they built more plants? Probably not! There you have regulations, cost overruns, and construction learning curves in a nutshell.
Safety
Nuclear regulations tend to emerge as responses to accidents, failures, and near misses. The company and NRC put together an after-action report, and new rules come out to make sure it never happens again. Deregulation isn't simple because almost every regulation has a real-life event behind it.
On the other hand, much of engineering is about tradeoffs. Changes to prevent one type of incident might increase risk elsewhere. The book gets thicker over time as Murphy's Law does its work and dependencies explode. There might be an opportunity to improve existing regulations to decrease costs and improve safety. The industry working groups and NRC want to do this, but it is a challenging engineering problem. Within any process, changing any variable impacts many others. Nuclear safety requires that engineers study every proposed change to the nth degree. No uncontrolled experiments are allowed.
There is a caricature of an evil industrialist that ignores safety. The other side has the absurdly strict regulator that kills everything without justification. Vastly underrated is the nuclear engineer working for a living that'd prefer not to be in the middle of a nuclear accident. Workers in the nuclear industry know politicians will close their plants if any accident or near-miss becomes a big event. Since the 1970s, all industrialized populations have shown zero tolerance for nuclear accidents, near misses, or releases, which is what ultimately drives the strict standards.
LWR designs, even passively cooled Gen III designs, require flawless support systems to maintain safety.
"New" Reactor Designs
Alternative reactor designs promise to be safer and offer higher efficiency. Salt/metal-cooled reactors and gas-cooled reactors are the two main paths to these goals.
Salts and metals have elevated boiling points that allow high operating temperatures at atmospheric pressure. The hot coolant heats a working fluid that runs a turbine. The reactor design can accommodate passive safety features that remove meltdown risk. The downside of salt is that it is very corrosive, and the downside of metals like sodium is flammability when exposed to air or water.
Gas-cooled reactors use gases like helium or CO2 that can be fed directly into a turbine. Gas-fed turbines tend to be smaller and more efficient than steam-fed condensing turbines. Some gas-cooled reactor concepts are over 50% efficient due to the high outlet temperature of the gas. Helium has the extra benefit of not becoming radioactive when cooling the reactor.
The fuel is typically meltdown-resistant smaller pellets in beds or arranged in blocks. Leaks are the downside of gas-cooled reactors. Gas can leak out, or water and other fluids can leak in. Early designs suffer from high operating costs as a result.
Prototypes and full-scale reactors using these technologies have been around for 60 years. Startups and researchers are trying these designs because they have higher ceilings than LWRs but extra challenges. New technology like better gas turbines makes some designs more attractive than 60 years ago.
Britain's Advanced Gas Reactor (AGR) is a cautionary tale. On paper, these reactors were cheaper than American and French LWRs but instead ended up being a boondoggle because of construction complexity and high operating costs. New reactor designs will likely need many iterations to reach their promise. They could be infeasible if regulators use the existing regulatory canon built on sixty years of LWR operation instead of allowing new tradeoffs that may be less expensive and safer.
Fusion?
Most fusion concepts are just a more complicated way to heat water. They fall victim to the heat engine problem and will not revolutionize human energy use.
The exception is the few startups that rethought generating electricity within their design, like Helion. Helion's cost goal is $10/MWh instead of $50/MWh because they don't need a traditional electricity generation setup.
If you ever wonder about fusion, ask: "How does this technology/company generate electricity?" You can stop reading if it looks like a traditional thermal power plant.
Traditional Nuclear Lobbyists Want You To Pay More
As we've seen, traditional LWRs have a cost problem. That is why the PR ignores costs or focuses only on operating costs.
Common Lines of Reasoning
-
Energy Return on Investment (EROI) of Nuclear Power Plants is Better
In the infancy of wind and solar, manufacturing them might use more energy than they produce. Improvements in technology mean this is no longer true. But PR folks can use old solar panel data while nuclear plants do well in the metric. The best part is they don't have to compare current solar electricity costs with today's nuclear power costs.
-
Nuclear Power Plants use Less Land
Nuclear power plants look very land efficient if you don't include the security buffer, the cooling water reservoir, or the no-fly zones imposed around them. It is not unusual to see nuclear advocates comparing land use of the reactor building against much less flattering metrics for wind or solar. For example, there might be eight wind turbines on 640 acres. The wind turbines and the roads might use 15 acres, but the comparison will show the wind farm using 640 acres of land.
-
Only Nuclear can Decarbonize
Nuclear was the only realistic way to decarbonize an electricity grid in the past. But nuclear costs increase rapidly after 50%-60% market share of generation. Plants require storage or exports because of their high fixed costs. Recently, places as diverse as Texas, California, Oklahoma/Iowa/Kansas/Nebraska (SPP), the UK, and Germany have rapidly decarbonized without nuclear. Most competitive grids in the US have tens of gigawatts of solar and storage in the interconnection queue. New technology like NetPower's zero-emission natural gas power plants can beat new combined-cycle plants on economics and decarbonize the last 10% of the electricity supply. All these technologies are more cost-effective and faster to build than new nuclear.
Prices are the best comparison because they account for energy use in manufacturing, construction time, and land use.
But what about nuclear running 24/7 while other technologies are variable?
Nuclear power plants produce 24/7 out of economic necessity to cover fixed costs. Many US nuclear plants are incapable of cycling power output up or down without changes to procedures or equipment. Laws and regulations also prevent automation like European plants use to change plant output. To load follow, plants would have to increase staffing to manage output changes. Costs increase to sell less power. Changing plant conditions is less safe than operating at steady conditions. The PR folks have done incredible work to spin a disadvantage into a perceived advantage. New coal and natural gas power plants have lower utilization by design because electricity demand changes dramatically during the day and through the seasons. Electricity from nuclear power plants becomes much more expensive as plant utilization falls.
Nuclear startup TerraPower opted to increase the capital cost of its design to add molten salt storage and oversize its power generation equipment. Its concept will store heat from the reactor in salt, then run the turbines harder in the afternoon when people want electricity. The reactor runs steady-state. Dispatchable production, whether enabled by storage or low fixed costs, is economically advantageous to generators that must run 24/7 to pay the bills.
Even if you think that natural gas should be banned or taxed away, nuclear will still end up uncompetitive. By the time you finish a single LWR, investors will build enough solar and storage to eat high daytime electricity rates. If you assume current nuclear plant operating costs and a very optimistic OCC then only a modest decline in cost makes solar + storage cheaper for 24/7 power. Solar and batteries have declined over 90% in price over the last decade, and the learning curve powering growth looks to continue.
Nuclear power must innovate to reduce fixed and capital costs to compete.
Political Economy of Existing Reactors and Regulation
So why aren't our politicians pushing to loosen up regulations?
-
Bad Incentives
If an accident were to happen and you advocated for less regulation, you take the blame whether it is justified or not.
-
Protecting Jobs
The opposite side of having high fixed costs is that nuclear power plants are money machines and job creators. Small towns with a nuclear power plant are rural oases. Representatives with plants in their district will fight to the death to keep the gravy train rolling. There are rich, anti-energy places, like California, that are exceptions.
-
Handouts are Easier than Reforms
Nuclear power plants owned by monopoly utilities can pass incremental costs on to consumers. Plants in competitive markets find it easier to lean on state legislatures for subsidies than attacking the iron triangle in Washington DC. Safely deregulating is difficult.
-
New Plants are Risky for Politicians and Utilities
Legislatures will punish utilities and their investors for massive cost overruns. Rapid rate increases do not make voters happy. The canceled VC Summer expansion in South Carolina and the overbudget Vogle project in Georgia are examples.
-
The Constituency for New Technology is Small
Politicians are the barriers to new technology, not NRC or the Department of Energy. Workers at places like Idaho National Lab favor new technology. NRC technical staff keep their heads down and follow the laws written by Congress. If NRC is too strict for the national interest, Congress needs to rewrite the law. For Congress to rewrite the law, they'd need a massive investment in engineering talent to work with NRC and the industry groups to do things like creating a new regulatory regime for Gen IV reactors. All the while, traditional nuclear contractors and interest groups oppose new laws that might help startups build better technology than the LWR.
Nuclear's Possible Futures
Investors Will Risk Capital for Better Technology
While new light water reactors are obsolete in competitive electricity markets in the US, startups are trying to find new niches.
The first group is "Small" Modular Reactor (SMR) startups. These reactors are between 70 MW and 250 MW instead of the typical 1000 MW LWR. The idea is that more construction can happen in a factory, compressing the schedule and reducing costs.
-
NuScale
NuScale is building a small, modular light water reactor. They bet that using a traditional design will ease regulatory approvals. The hope is that indoor manufacturing paired with incremental improvements will provide enough cost reduction to be competitive in some applications. They aren't even using more enriched fuel like most startups. True to form, they already have some licensing done but won't have a commercial plant before 2030.
-
TerraPower
TerraPower is building a sodium-cooled fast reactor. TerraPower's reactor will be more efficient than LWRs and has attached storage. TerraPower will not be producing power before 2030.
The more radical challengers are attempting to build microreactors for off-grid markets, competing with diesel generators.
-
Oklo
Oklo wanted to have its first commercial reactor operating by 2024. The company recently suffered a major regulatory setback when NRC rejected its application for lack of information but invited Oklo to try again later. Their design is a salt-cooled fast reactor that uses carbon dioxide as a turbine working fluid instead of water. CO2 thermodynamic cycles are more efficient with more compact equipment, and CO2 does not react violently with sodium. Large-scale applications of CO2 turbines are relatively new, with NetPower's 50 MW natural gas power plant in Texas being one of the first.
Oklo's reactor requires zero workers on site, and instead of being refueled every 12-18 months, it can go over five years and use the nuclear waste from LWRs instead of newly mined uranium. The company is hoping for construction times of around a year. Security and safety come from the meltdown-resistant fuel buried in the ground. Any problems would give the local SWAT team time to respond or workers to intervene. The entire concept makes a concerted effort to decrease fixed costs. Reactor size can increase over time to compete in on-grid markets.
-
Radiant
Radiant is building a helium-cooled reactor that can fit in a shipping container. They do not specify what heat engine design they use, but it makes sense that they will feed hot helium directly to a turbine. Their aspirational schedule is a few years behind Oklo. Radiant's regulatory strategy appears to be similar to Oklo, so Oklo's troubles will likely impact Radiant as well.
Few Evolutionary Paths are Available
If we put all this knowledge together, few easy paths for nuclear growth emerge.
One path is that the nuclear industry finds a way for higher prices to be politically palatable. The government could subsidize a new wave of LWRs. Hopefully, they are built with a consistent design at a steady pace in pairs to limit the bill to the taxpayer and utility customers. Call it the "East Asia Plan."
The other path is that entrepreneurs and regulators get new technologies across the finish line of winning market competitions. We know that new technology resets learning curves for nuclear plants. So while the nuclear industry needs new technology to be competitive, it can't go straight into the teeth of PJM or ERCOT. Microreactors competing with diesel generators is a very logical starting point. The cost of the electricity is high enough to justify the pain of iterating through early models. As designs and techniques improve, the reactors might be competitive in more markets. It sounds good on paper, but nuclear is hard, especially when the standards for safety and reliability are high. Viability requires fast iterations because competing technologies aren't standing still. And the more nuclear regulators or other environmental regulations slow the process down, the lower the probability of success is. Given NRC's recent action with Oklo, the entrepreneurial path seems grim.
China
Even China has slowed its LWR ambitions following recent industry turmoil with over-budget projects and bankruptcies.
China also has aspirations for high-temperature gas-cooled reactors and sodium-cooled fast reactors. Two state-owned enterprises are the primary developers. They may not have the urgency to reach practical designs.
Where Nuclear Can Always Win
Josh Hall lays out the ultimate nuclear case in his book "Where is My Flying Car?" Actual nuclear fuel is incredibly energy-dense, holding orders of magnitude more energy per unit of mass or volume than liquid fuels or batteries. It is dirt cheap on a total energy basis.
Because of the heat engine problem, our ability to harness the fuel is lacking. In the future, we might invent betavoltaic batteries with better power density or better solid-state heat engines like thermoelectric generators. Instead of competing to provide bulk electricity, nuclear could power flying cars or appliances in a way no other energy sources can match. Miniaturized nuclear power is the ultimate energy source.
Pay to Relive the Past or Forge Ahead?
Should we have built more nuclear plants in the 60s and 70s when coal was the primary alternative? Almost certainly. It may not makes sense to build new LWRs today, but we can reduce how much existing plants cost to operate and fast-track new technologies to the market through better regulation.
Nuclear power requires considerable effort to catch up and keep pace with competitors. New light water reactors are dead on arrival without governments providing low-interest loans or giving utility companies monopoly powers to charge higher rates. Incremental SMRs that won't be available for another decade risk coming online too late and too expensive as solar, wind, and battery prices continue to fall. Micro reactors with accelerated schedules have a better chance of breaking into the mainstream given their low fixed cost potential and faster iteration, but only if we try to build them.
Nuclear advocates will have to convince consumers and taxpayers to pay significantly more for electricity unless they reduce the cost of the technology. There is a good chance nuclear does not have a true revival until we miniaturize it.
-
Lazard LCOE Analysis
-
Lovering, Yip, Nordhaus Historical Nuclear Costs
-
Breakdown of Nuclear OCC Cost Components
-
Construction Physics on Construction Learning Curves
-
Vogle Cable Tray Separation Problems
-
If you think a candle touching off a critical safety event sounds ridiculous, the third-worst US nuclear safety event was started by a worker changing a lightbulb and accidentally dropping it. The lightbulb shorted a control system that led to a cascade of failures.
Why is the Nuclear Power Industry Stagnant?
2022 January 10 Twitter Substack See all postsThanks to Bill and Sharon for answering all my nuclear engineering questions over the years, even when the nuclear plant was the last thing they wanted to think about. Any mistakes are my own.
Nuclear power plants produce around 20% of US electricity and the majority in France. Yet the industry is stagnant. Let's look at why.
Examining Cost
Operating cost matters for existing, depreciated nuclear power plants. Construction costs determine if investors will fund new power plants.
Operating Cost
Nuclear power plants have low fuel costs, meaning a large portion of their operating cost is fixed. Instead of buying fuel regularly like a coal or natural gas power plant, traditional civil nuclear plants refuel every 12-18 months. Nuclear fuel cycles create incentives similar to fixed costs.
Lazard estimates that a nuclear plant's operating costs vary between $28/MWh and $30/MWh.[1] Fuel is only 1/3 of that cost. A nuclear power plant has fixed operating costs 8x larger per kW than a natural gas combined cycle power plant and 2-3x a coal-fired power plant. Higher fixed costs come from a workforce that nuclear plants require to satisfy regulators, maintain higher safety standards, and manage the extra complexity nuclear technology brings. Operating costs would fall to ~$20/MWh if assuming a rate closer to coal power plants (which have similar power generating equipment). While existing plants likely suffer too much regulation, traditional nuclear power plant operation is more complex than a coal or gas plant. The plants would require more engineering talent and overhead even with zero regulation.
When I analyzed the largest US electricity market, PJM, for my solar post, I found that in 2019 and 2020, wholesale prices were only averaging around $25/MWh due to cheap natural gas. Even a completely paid-off nuclear plant can barely make ends meet in most US electricity markets. Existing plants in deregulated markets need their entire regulatory burden removed to stay open. There is a reason plants have been closing, or state legislatures are scrambling to work out subsidy deals.
The situation will deteriorate further. Daylight electricity prices in PJM averaged $29/MWh. Lazard estimates that new, unsubsidized utility-scale solar can compete at $30/MWh and as low as $25/MWh with the federal tax credit. New solar will lower daytime electricity prices, dropping average wholesale prices further. PJM is one of the worst markets for solar, and it still has almost 30 GW of solar applying for interconnection. Once solar is online, its total operating costs are under $5/MWh.
Traditional, depreciated nuclear power plants barely break even with a lighter regulatory burden. Expensive markets, like California ISO, are hostile to nuclear because opposition to energy is stiff.
Construction Cost
Traditional nuclear power plants are expensive to build. Historical costs range from <$1000/kW to infinite in the case of construction planning disasters like the attempted VC Summer plant expansion. Lazard estimates $8000-$12,000/kW based on plants under construction in the US, UK, and France.
Pecularities of Nuclear CAPEX Estimates
Dollars per kilowatt are the units for nuclear power plant construction costs. Numbers do not include the full scope of costs and are known as Overnight Construction Cost (OCC). OCC does not include interest payments during construction or decommissioning. Since governments often subsidize decommissioning and interest rates for nuclear power plants, OCC is better for comparison purposes.
Interest payments during construction can account for a large portion of upfront costs. The interest accounts for 12% of construction costs for a plant built in five years with a five percent interest rate. But if construction extends to 10 years with a 15% interest rate, the payments are more than the OCC.
Any OCC number is incomplete in understanding nuclear power economics.
Historical Costs
Nuclear power plant cost varies dramatically by country and period. Lovering, Yip, and Nordhaus compiled historical OCC data in their 2016 paper.[2]
Lovering, Yip, Nordhaus
The first demonstration plants were expensive. Prices fell initially from scaling effects. Developers built many early nuclear plants in the US on underbid fix-priced contracts to establish their business. The pricing was not sustainable because of the underbidding, and these stripped-down designs were underwhelming in safety and reliability. The US and other markets saw cost increases as designers added safety and reliability features. US costs skyrocketed after Three Mile Island, while most other countries saw milder increases. A few countries like South Korea have embarked on building programs that utilize best practices to return near $2000/kW.
With experienced construction teams and engaged regulators, overnight construction costs for new plants can come in under $2000/kWh. Inexperienced construction teams and out-of-touch regulators can inflate the price by five times (or infinitely). The US, France, India, West Germany, and South Korea have seen periods of $2000/kW construction costs. Only India and South Korea have managed this feat recently.
Today's Competitive Landscape
Even when costs are low, construction times are long. Pre-Three Mile Island US nuclear plants had median construction times over five years. France saw similar timelines for its buildout. New plants are in countries where government-subsidized low-interest loans improve economics.
Lovering, Yip, Nordhaus
Lovering, Yip, Nordhaus
Technologies with low construction intensity like solar, wind, and natural gas turbines dominate the new power plant market. A $2000/kW plant built in under six years would equate to $34/MWh in capital costs. A low regulatory burden nuclear power plant would require over $54/MWh to break even. With interest-free loans, the total still comes to $46/MWh. Even if you could build a reactor with low-interest loans, government-subsidized decommissioning, plus 1960s regulation and labor costs, you'd still go bankrupt in competitive markets.
Learning Curves to the Rescue?
Manufacturing learning curves have led to dramatic falls in battery, solar panel, and wind turbine prices. Can nuclear see the same?
The Heat Engine Problem
Heat engines convert heat to work, like turning a generator. Heat engines can never be 100% efficient. The greater the temperature differential between the hot fluid and the cold fluid, the better efficiency is.
Physical layout of the Rankine cycle 1. Pump, 2. Boiler, 3. Turbine, 4. Condenser. Q = Heat and W = Work. Source: Andrew Ainsworth
Traditional thermal power plants, including nuclear and coal, heat water to make steam. The steam powers a turbine that turns a generator, producing electricity. The equipment and operation of the steam cycle are inherently expensive compared to gas turbines or solid-state technologies like solar panels. Hence, the heat engine problem.
Light Water Reactors
Most nuclear power plants are in a design family known as Light Water Reactors (LWR). The designs use water to cool the reactor and as a working fluid to drive a turbine. The reactor portion of the plant is only ~25% of construction costs.[3]
The rest of the facility is typical power plant components like heat exchangers, pumps, cooling towers, turbines, generators, etc. Nuclear plants have requirements like cobalt-free components, but other processing industries have similar limitations. These technologies are already far down a learning curve measured in hundreds of years, limiting potential cost declines.
LWRs have low efficiency, typically between 30% and 35%. A modern combined-cycle natural gas power plant is over 60% efficient. Low efficiency means more equipment and workers to produce the same amount of electricity. Improving LWR efficiency requires higher steam temperatures and pressures.
For the two main LWR designs, pressurized water reactors (PWR) and boiling water reactors (BWR), basic materials technology and economics limit the pressure and temperature of steam the plant can make. While fossil power plants often use superheated steam that allows more efficient turbine operation, PWRs and BWRs typically use saturated or slightly superheated steam.
The steam is saturated by definition in BWRs because it boils in the reactor vessel. BWRs run steam directly from the reactor to the turbine, meaning the turbine, condenser, and feedwater systems become irradiated. PWRs have a pressurized water loop cooling the reactor that transfers heat by boiling clean water in a heat exchanger known as a steam generator. Only the loop water becomes radioactive. PWRs can superheat steam, but the economics of the steam generator limit it. Boiling water has ten times the heat transfer of superheated steam, requiring a dramatically larger heat exchanger to superheat. In a fossil plant boiler, the firing temperature of the coal, gas, or oil might be hundreds of degrees higher than the steam. Because PWRs use pressure to prevent reactor loop water from boiling, the temperature differential is much tighter. Heat transfer increases with temperature differential, so the superheated steam heat transfer penalty for fossil-fired plants is lower.
Source
If better efficiency isn't realistic, what is available? Longer fuel cycles can help, but the government places legal limits on the enrichment of LWR fuel for safety and proliferation reasons. Some new designs use natural circulation within the reactor loop water, providing some savings. Data from the Lovering paper suggests that standardizing a plant design, building plants in pairs, and having a consistent regulatory regime can lower costs, like in S. Korea.
In a post for his Construction Physics blog, Brian Potter did a deep dive into learning curves in the construction industry.[4] Learning curves exist, but they are reset by any design change, moving to a new site, or changing crews. The findings closely match what Lovering, Yip, and Nordhaus found drove cost reduction or escalation. It is unlikely there is a steep learning curve for LWRs below historical costs. Design and regulatory consistency paired with multiple copies at one site can mitigate cost inflation.
An anecdote from the massively overbudget Vogle Reactors 3 and 4 illuminates how inexperience interacts with regulations and safety requirements. In 1974, a candle started an insulation fire in the Brown's Ferry Nuclear Plant, burning a cable tray. [6] Operators prevented a meltdown, but independent safety systems went offline because their control wiring shared a cable tray. If one fire can disable redundant safety mechanisms then they aren't redundant. Cable tray separation of critical safety systems has been a core principle of nuclear safety since and came before Three Mile Island in 1979.
A Cable Tray Source: Duraline
In 2021, the Nuclear Regulatory Commission (NRC) dinged Southern Company for over 600 cable separation discrepancies. [5] Southern has to pay to fix it and pay interest on the loans through the delays. It is horrifically expensive since so many cables are in a tray. Is this regulation costly and lengthening construction timelines? Yes. Is this regulation based on the history of operating power plants safely? Yes. Would Southern repeat this mistake if they built more plants? Probably not! There you have regulations, cost overruns, and construction learning curves in a nutshell.
Safety
Nuclear regulations tend to emerge as responses to accidents, failures, and near misses. The company and NRC put together an after-action report, and new rules come out to make sure it never happens again. Deregulation isn't simple because almost every regulation has a real-life event behind it.
On the other hand, much of engineering is about tradeoffs. Changes to prevent one type of incident might increase risk elsewhere. The book gets thicker over time as Murphy's Law does its work and dependencies explode. There might be an opportunity to improve existing regulations to decrease costs and improve safety. The industry working groups and NRC want to do this, but it is a challenging engineering problem. Within any process, changing any variable impacts many others. Nuclear safety requires that engineers study every proposed change to the nth degree. No uncontrolled experiments are allowed.
There is a caricature of an evil industrialist that ignores safety. The other side has the absurdly strict regulator that kills everything without justification. Vastly underrated is the nuclear engineer working for a living that'd prefer not to be in the middle of a nuclear accident. Workers in the nuclear industry know politicians will close their plants if any accident or near-miss becomes a big event. Since the 1970s, all industrialized populations have shown zero tolerance for nuclear accidents, near misses, or releases, which is what ultimately drives the strict standards.
LWR designs, even passively cooled Gen III designs, require flawless support systems to maintain safety.
"New" Reactor Designs
Alternative reactor designs promise to be safer and offer higher efficiency. Salt/metal-cooled reactors and gas-cooled reactors are the two main paths to these goals.
Salts and metals have elevated boiling points that allow high operating temperatures at atmospheric pressure. The hot coolant heats a working fluid that runs a turbine. The reactor design can accommodate passive safety features that remove meltdown risk. The downside of salt is that it is very corrosive, and the downside of metals like sodium is flammability when exposed to air or water.
Gas-cooled reactors use gases like helium or CO2 that can be fed directly into a turbine. Gas-fed turbines tend to be smaller and more efficient than steam-fed condensing turbines. Some gas-cooled reactor concepts are over 50% efficient due to the high outlet temperature of the gas. Helium has the extra benefit of not becoming radioactive when cooling the reactor.
The fuel is typically meltdown-resistant smaller pellets in beds or arranged in blocks. Leaks are the downside of gas-cooled reactors. Gas can leak out, or water and other fluids can leak in. Early designs suffer from high operating costs as a result.
Prototypes and full-scale reactors using these technologies have been around for 60 years. Startups and researchers are trying these designs because they have higher ceilings than LWRs but extra challenges. New technology like better gas turbines makes some designs more attractive than 60 years ago.
Britain's Advanced Gas Reactor (AGR) is a cautionary tale. On paper, these reactors were cheaper than American and French LWRs but instead ended up being a boondoggle because of construction complexity and high operating costs. New reactor designs will likely need many iterations to reach their promise. They could be infeasible if regulators use the existing regulatory canon built on sixty years of LWR operation instead of allowing new tradeoffs that may be less expensive and safer.
Fusion?
Most fusion concepts are just a more complicated way to heat water. They fall victim to the heat engine problem and will not revolutionize human energy use.
The exception is the few startups that rethought generating electricity within their design, like Helion. Helion's cost goal is $10/MWh instead of $50/MWh because they don't need a traditional electricity generation setup.
If you ever wonder about fusion, ask: "How does this technology/company generate electricity?" You can stop reading if it looks like a traditional thermal power plant.
Traditional Nuclear Lobbyists Want You To Pay More
As we've seen, traditional LWRs have a cost problem. That is why the PR ignores costs or focuses only on operating costs.
Common Lines of Reasoning
Energy Return on Investment (EROI) of Nuclear Power Plants is Better
In the infancy of wind and solar, manufacturing them might use more energy than they produce. Improvements in technology mean this is no longer true. But PR folks can use old solar panel data while nuclear plants do well in the metric. The best part is they don't have to compare current solar electricity costs with today's nuclear power costs.
Nuclear Power Plants use Less Land
Nuclear power plants look very land efficient if you don't include the security buffer, the cooling water reservoir, or the no-fly zones imposed around them. It is not unusual to see nuclear advocates comparing land use of the reactor building against much less flattering metrics for wind or solar. For example, there might be eight wind turbines on 640 acres. The wind turbines and the roads might use 15 acres, but the comparison will show the wind farm using 640 acres of land.
Only Nuclear can Decarbonize
Nuclear was the only realistic way to decarbonize an electricity grid in the past. But nuclear costs increase rapidly after 50%-60% market share of generation. Plants require storage or exports because of their high fixed costs. Recently, places as diverse as Texas, California, Oklahoma/Iowa/Kansas/Nebraska (SPP), the UK, and Germany have rapidly decarbonized without nuclear. Most competitive grids in the US have tens of gigawatts of solar and storage in the interconnection queue. New technology like NetPower's zero-emission natural gas power plants can beat new combined-cycle plants on economics and decarbonize the last 10% of the electricity supply. All these technologies are more cost-effective and faster to build than new nuclear.
Prices are the best comparison because they account for energy use in manufacturing, construction time, and land use.
But what about nuclear running 24/7 while other technologies are variable?
Nuclear power plants produce 24/7 out of economic necessity to cover fixed costs. Many US nuclear plants are incapable of cycling power output up or down without changes to procedures or equipment. Laws and regulations also prevent automation like European plants use to change plant output. To load follow, plants would have to increase staffing to manage output changes. Costs increase to sell less power. Changing plant conditions is less safe than operating at steady conditions. The PR folks have done incredible work to spin a disadvantage into a perceived advantage. New coal and natural gas power plants have lower utilization by design because electricity demand changes dramatically during the day and through the seasons. Electricity from nuclear power plants becomes much more expensive as plant utilization falls.
Nuclear startup TerraPower opted to increase the capital cost of its design to add molten salt storage and oversize its power generation equipment. Its concept will store heat from the reactor in salt, then run the turbines harder in the afternoon when people want electricity. The reactor runs steady-state. Dispatchable production, whether enabled by storage or low fixed costs, is economically advantageous to generators that must run 24/7 to pay the bills.
Even if you think that natural gas should be banned or taxed away, nuclear will still end up uncompetitive. By the time you finish a single LWR, investors will build enough solar and storage to eat high daytime electricity rates. If you assume current nuclear plant operating costs and a very optimistic OCC then only a modest decline in cost makes solar + storage cheaper for 24/7 power. Solar and batteries have declined over 90% in price over the last decade, and the learning curve powering growth looks to continue.
Nuclear power must innovate to reduce fixed and capital costs to compete.
Political Economy of Existing Reactors and Regulation
So why aren't our politicians pushing to loosen up regulations?
Bad Incentives
If an accident were to happen and you advocated for less regulation, you take the blame whether it is justified or not.
Protecting Jobs
The opposite side of having high fixed costs is that nuclear power plants are money machines and job creators. Small towns with a nuclear power plant are rural oases. Representatives with plants in their district will fight to the death to keep the gravy train rolling. There are rich, anti-energy places, like California, that are exceptions.
Handouts are Easier than Reforms
Nuclear power plants owned by monopoly utilities can pass incremental costs on to consumers. Plants in competitive markets find it easier to lean on state legislatures for subsidies than attacking the iron triangle in Washington DC. Safely deregulating is difficult.
New Plants are Risky for Politicians and Utilities
Legislatures will punish utilities and their investors for massive cost overruns. Rapid rate increases do not make voters happy. The canceled VC Summer expansion in South Carolina and the overbudget Vogle project in Georgia are examples.
The Constituency for New Technology is Small
Politicians are the barriers to new technology, not NRC or the Department of Energy. Workers at places like Idaho National Lab favor new technology. NRC technical staff keep their heads down and follow the laws written by Congress. If NRC is too strict for the national interest, Congress needs to rewrite the law. For Congress to rewrite the law, they'd need a massive investment in engineering talent to work with NRC and the industry groups to do things like creating a new regulatory regime for Gen IV reactors. All the while, traditional nuclear contractors and interest groups oppose new laws that might help startups build better technology than the LWR.
Nuclear's Possible Futures
Investors Will Risk Capital for Better Technology
While new light water reactors are obsolete in competitive electricity markets in the US, startups are trying to find new niches.
The first group is "Small" Modular Reactor (SMR) startups. These reactors are between 70 MW and 250 MW instead of the typical 1000 MW LWR. The idea is that more construction can happen in a factory, compressing the schedule and reducing costs.
NuScale
NuScale is building a small, modular light water reactor. They bet that using a traditional design will ease regulatory approvals. The hope is that indoor manufacturing paired with incremental improvements will provide enough cost reduction to be competitive in some applications. They aren't even using more enriched fuel like most startups. True to form, they already have some licensing done but won't have a commercial plant before 2030.
TerraPower
TerraPower is building a sodium-cooled fast reactor. TerraPower's reactor will be more efficient than LWRs and has attached storage. TerraPower will not be producing power before 2030.
The more radical challengers are attempting to build microreactors for off-grid markets, competing with diesel generators.
Oklo
Oklo wanted to have its first commercial reactor operating by 2024. The company recently suffered a major regulatory setback when NRC rejected its application for lack of information but invited Oklo to try again later. Their design is a salt-cooled fast reactor that uses carbon dioxide as a turbine working fluid instead of water. CO2 thermodynamic cycles are more efficient with more compact equipment, and CO2 does not react violently with sodium. Large-scale applications of CO2 turbines are relatively new, with NetPower's 50 MW natural gas power plant in Texas being one of the first.
Oklo's reactor requires zero workers on site, and instead of being refueled every 12-18 months, it can go over five years and use the nuclear waste from LWRs instead of newly mined uranium. The company is hoping for construction times of around a year. Security and safety come from the meltdown-resistant fuel buried in the ground. Any problems would give the local SWAT team time to respond or workers to intervene. The entire concept makes a concerted effort to decrease fixed costs. Reactor size can increase over time to compete in on-grid markets.
Radiant
Radiant is building a helium-cooled reactor that can fit in a shipping container. They do not specify what heat engine design they use, but it makes sense that they will feed hot helium directly to a turbine. Their aspirational schedule is a few years behind Oklo. Radiant's regulatory strategy appears to be similar to Oklo, so Oklo's troubles will likely impact Radiant as well.
Few Evolutionary Paths are Available
If we put all this knowledge together, few easy paths for nuclear growth emerge.
One path is that the nuclear industry finds a way for higher prices to be politically palatable. The government could subsidize a new wave of LWRs. Hopefully, they are built with a consistent design at a steady pace in pairs to limit the bill to the taxpayer and utility customers. Call it the "East Asia Plan."
The other path is that entrepreneurs and regulators get new technologies across the finish line of winning market competitions. We know that new technology resets learning curves for nuclear plants. So while the nuclear industry needs new technology to be competitive, it can't go straight into the teeth of PJM or ERCOT. Microreactors competing with diesel generators is a very logical starting point. The cost of the electricity is high enough to justify the pain of iterating through early models. As designs and techniques improve, the reactors might be competitive in more markets. It sounds good on paper, but nuclear is hard, especially when the standards for safety and reliability are high. Viability requires fast iterations because competing technologies aren't standing still. And the more nuclear regulators or other environmental regulations slow the process down, the lower the probability of success is. Given NRC's recent action with Oklo, the entrepreneurial path seems grim.
China
Even China has slowed its LWR ambitions following recent industry turmoil with over-budget projects and bankruptcies.
China also has aspirations for high-temperature gas-cooled reactors and sodium-cooled fast reactors. Two state-owned enterprises are the primary developers. They may not have the urgency to reach practical designs.
Where Nuclear Can Always Win
Josh Hall lays out the ultimate nuclear case in his book "Where is My Flying Car?" Actual nuclear fuel is incredibly energy-dense, holding orders of magnitude more energy per unit of mass or volume than liquid fuels or batteries. It is dirt cheap on a total energy basis.
Because of the heat engine problem, our ability to harness the fuel is lacking. In the future, we might invent betavoltaic batteries with better power density or better solid-state heat engines like thermoelectric generators. Instead of competing to provide bulk electricity, nuclear could power flying cars or appliances in a way no other energy sources can match. Miniaturized nuclear power is the ultimate energy source.
Pay to Relive the Past or Forge Ahead?
Should we have built more nuclear plants in the 60s and 70s when coal was the primary alternative? Almost certainly. It may not makes sense to build new LWRs today, but we can reduce how much existing plants cost to operate and fast-track new technologies to the market through better regulation.
Nuclear power requires considerable effort to catch up and keep pace with competitors. New light water reactors are dead on arrival without governments providing low-interest loans or giving utility companies monopoly powers to charge higher rates. Incremental SMRs that won't be available for another decade risk coming online too late and too expensive as solar, wind, and battery prices continue to fall. Micro reactors with accelerated schedules have a better chance of breaking into the mainstream given their low fixed cost potential and faster iteration, but only if we try to build them.
Nuclear advocates will have to convince consumers and taxpayers to pay significantly more for electricity unless they reduce the cost of the technology. There is a good chance nuclear does not have a true revival until we miniaturize it.
Lazard LCOE Analysis
Lovering, Yip, Nordhaus Historical Nuclear Costs
Breakdown of Nuclear OCC Cost Components
Construction Physics on Construction Learning Curves
Vogle Cable Tray Separation Problems
If you think a candle touching off a critical safety event sounds ridiculous, the third-worst US nuclear safety event was started by a worker changing a lightbulb and accidentally dropping it. The lightbulb shorted a control system that led to a cascade of failures.