Why Gas is Useful
Efficient, Cheap Power Plants
Gas power plants are cheap.
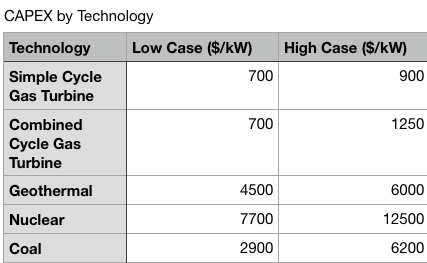
Source: Lazard
Why are gas plants so cheap? They have less equipment. A gas turbine takes in compressed gas and air, burns it, spits out the exhaust, and turns a generator. Modern turbines are as efficient as coal or nuclear plants and a fraction of the size of hulking steam turbines. They are 3x as efficient as the average geothermal facility.
Coal, nuclear, and geothermal plants utilize more complex thermodynamic cycles. They have a boiler, a steam turbine, a generator, a condenser, a boiler feed water pump, more cooling towers, and a water purification system for boiler feed water. Coal plants contend with solids handling, and nuclear plants have complex reactors.
Combined cycle natural gas power plants use hot exhaust from the turbine to make steam like traditional thermal plants. About 2/3 of plant output comes from the gas turbine and 1/3 from the steam turbine. Total efficiency can be over 60% with less equipment and labor than boiler-style thermal plants.
Cheap Storage
Most natural gas storage is in depleted reservoirs. They fill up in the summer when gas demand is low and empty in the winter when gas demand is high.
Building a depleted reservoir gas storage facility costs about $6 million per billion cubic feet of gas. That equates to $0.02/kWh. A grid storage lithium-ion battery currently costs $250-$300/kWh.
The marginal cost of storing gas is determined by renting space in the storage facility and compressing it into the reservoir, usually ~$0.50/MCF for a season. Spot natural gas prices have been between $1.50 and $6 over the last decade. Efficiency determines the marginal cycle cost for a battery. Most lithium-ion battery systems are 90%+ efficient. Tesla's Powerwall has a 92.5% round trip efficiency.
Reservoir permeability limits gas injection/discharge rates to emptying once per season. Salt dome storage facilities are an exception that can cycle faster but have higher construction costs. Batteries can cycle within a few hours.
Batteries have an advantage in short-term storage, while natural gas storage is much better for long-duration storage.
Better Than X
Hydrogen
Why not store hydrogen instead of natural gas?
Hydrogen embrittles metal that pipelines and storage facility wells are made of, limiting usage in existing infrastructure.
Methane (the primary molecule in natural gas) has three times the energy per volume as hydrogen. A switch to hydrogen would mean we'd need three times more pipeline, compressor, and gas storage capacity.
Hydrogen is more expensive to store because of poor volumetric energy density, and it needs all new infrastructure. If we see widespread hydrogen storage, it will likely be local and only for industrial and electricity use. Building new interstate pipelines is increasingly difficult. As we've seen with electricity transmission, critics do not make an exception for "green" projects. I will remain skeptical.
Better Than Air
Compressed air storage also uses caverns and reservoirs as cheap, long-term storage. Compressed methane is ~80x more energy-dense than compressed air. Facilities need new turbines and grid connections, unlike natural gas.
Ammonia
Ammonia is easy to liquefy, so it has good volumetric energy density. Proponents favor applications like marine fuel and gas turbines.
The downsides are that it is poisonous, burns slow, releases tons of NOx when burned, and is less dense than regular ship fuel. The toxic aspect eliminates its use in residential applications. The combustion characteristics mean turbines need larger combustion chambers and more emissions control than natural gas turbines. And the lower volumetric density means it is vulnerable to drop-in synthetic liquid fuels in maritime applications.
Pumped Hydro and Other Cats and Dogs
Pumped hydro costs a thousand times more per unit of energy than natural gas storage. All the other random pet technologies are expensive, too. Storing energy in hydrocarbons is laughably cheap. Competing technologies tend to be awkward tweeners. They can't compete against batteries in short-term storage or against gas in longer-duration storage.
Electric Home Heating
A previous post covers why it is best to let markets sort out heating fuel rather than mandating electrification. Heating can require massive amounts of energy in a short time. Natural gas handles this volatility better than electric grids.
If utilities charge households correctly for electricity use, electric heating looks less economical in many places. Residential customers usually only pay for the kilowatt-hours they use. Costs like distribution, transmission, and building power plants are all rolled in. It is much cheaper for utilities to serve a customer that uses electricity consistently than one that uses the same total amount at sporadic rates. Large power users pay for each kilowatt-hour and a "demand" fee based on their peak usage. Demand charges might be half a big user's bill. Non-electric heat customers subsidize customers with electric heating who see power spikes on a few cold days a year. Grid stress increases because the utility didn't collect demand charges to build out low utilization power plants and transmission lines.
The dynamic can flip in hot places where cold snaps are non-existent. The gas heat home uses electricity at a high rate in the summer with low usage in winter. The electric heat home raises the utilization of the system without requiring new investment.
When markets send the correct signals, they maximize reliability and minimize costs.
Dispatchable
Zero carbon resources like geothermal, nuclear, solar, nuclear, and wind have mostly fixed costs. Upfront costs are high, and variable cost is low.
Coal, natural gas, and hydro (usually) are dispatchable. Gas and coal have lower fixed costs and higher marginal (fuel) costs, while limited hydropower reservoir levels add opportunity costs.
Geothermal and nuclear may technically be dispatchable, but it is expensive. Running in load-following mode decreases revenue and adds costs by increasing labor and maintenance. Running a nuclear or geothermal plant at 50% capacity more than doubles the cost of electricity it produces. Like solar, wind, and batteries, their CAPEX must fall even further to increase market share.
The average peaker only runs at about 11% of its full utilization. Replacing peakers with 24/7 power sources like nuclear and geothermal would increase their cost per megawatt-hour by roughly 10x. The fact that natural gas peakers already exist makes the economics for competitors even worse. Adding batteries and renewables can extend the life of existing gas peaking plants because instead of starting every evening to run for an hour, they run in longer spurts when demand and prices are high. Turbine maintenance and lifetime are both impacted by cycles run.
While we may imagine our electricity use to be steady, variance is high. There is only so much room for nuclear or geothermal before gas turbines, batteries, or import/export capability are much cheaper than continuing to add nuclear or geothermal capacity. It ends up that sources like solar and nuclear are closer substitutes than commonly assumed. Only grids with high hydro penetration can do without gas or coal at a reasonable cost.

Demand changes a lot even in short periods

Gas does the hard work of matching supply and demand. Source: EIA
There are ideas like powering existing coal plant steam turbines with hot, dry rock geothermal steam that may have a chance to nose in. The challenge is still CAPEX. Even with the bluest sky optimistic assumptions, it would take $10/MCF and $20/MCF gas prices to put a retrofit facility in the green against existing simple cycle turbines and combined-cycle plants at 10% capacity factors. There might not be any coal plants to retrofit once the technology and a sufficient carbon tax exist. Or competing technologies like synthetic natural gas or cheap carbon capture could swoop in under $10/MCF. It may be a better option for places like Asia with higher gas prices, fewer existing gas plants, and a surfeit of coal plants.
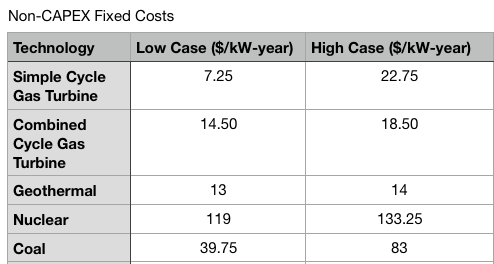
Source: Lazard
Natural Gas Power Plant Inventory
Natural gas power plants make up 43% of US electricity generation capacity. About half of that capacity is relatively new combined-cycle plants. Gas turbines and a raft of gas-fired steam turbines make up the rest.
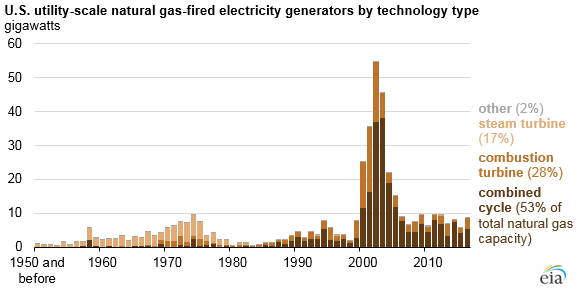
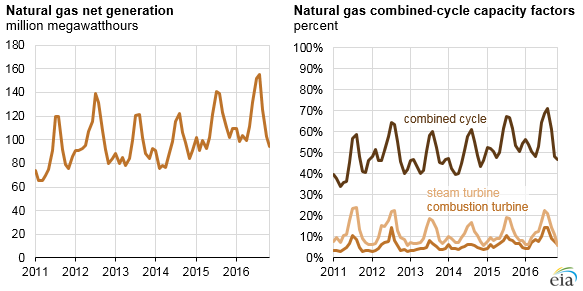
Source: EIA
Combined cycle plants shoulder most of the burden matching daily and seasonal electricity demand patterns. The steam and gas turbine peaker plants see most of their use on summer afternoons and evenings. Gas-fired steam turbine plants are usually more efficient than older gas turbines which can be as low as 20% efficiency. New turbines can be as high as 35% efficient. It is hard to kill depreciated plants in low-utilization applications.
Gas power plants compete directly with coal power plants in providing baseload power and meeting peaking/seasonal demand. Coal-to-Gas switching is one of the relief valves for balancing gas supply and demand. When prices are high, coal burns more, and when prices are low, gas burn increases. Utilities are closing most coal power plants because they are older, have higher fixed costs, and environmental compliance is more expensive.
As renewables increase market share, coal will decrease while combined cycle plants move towards seasonal and peaking duty. These plants can survive long past their estimated service date because of low fixed costs. Solar and batteries can meet typical afternoon/evening peaks cost-effectively. Gas power plants might run more of the day when sun or wind resources are less available instead of running for a few hours on hot afternoons. Natural gas prices might become more volatile, but fuel is a smaller share of costs in applications like home heating and peak electricity generation.
It is funny when pundits criticize renewables for needing backup generation when they already pay for such a system every month on their electric bill.
How Much Gas Do We Need?
The National Renewable Energy Laboratory recently released a study showing market share based on various scenarios.
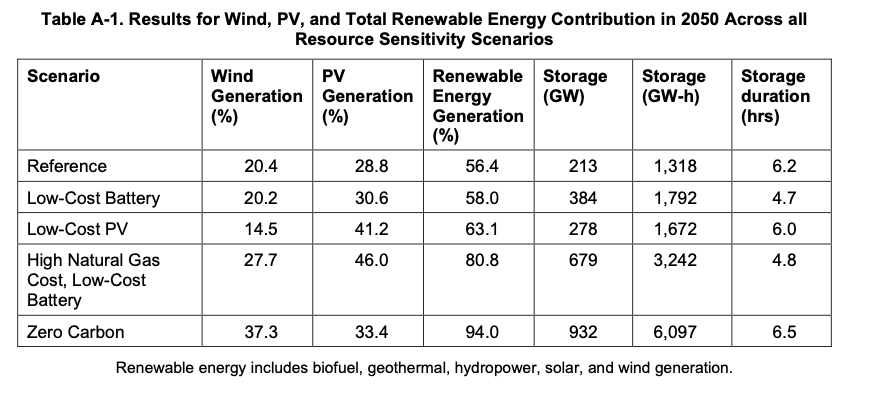
Cheap Solar = $15/MWh, Cheap Batteries = $80/kWh, Baseline Gas = $3.50/MCF, Expensive Gas = $6.50/MCF Source: NREL
Even with conservative assumptions about cost declines and assuming natural gas prices at $3.50/MCF, non-nuclear renewables will make up a large share of our grid by 2050. Every solar panel and battery added to the grid sees lower utilization, requiring cost decreases for higher penetration. Assuming 20% nuclear generation market share, 83% of our electricity would be zero-carbon even with cheap gas.
Adding low utilization renewables and batteries become more competitive if natural gas prices are high. High is ~$6.50/MCF in this example. That price is incredibly low for Europe and Asia and is not historically high for the US. Grid battery storage doubles to meet the new demand in this scenario. Using zero gas requires doubling storage again and a massive buildout of wind and long-distance transmission (yikes!) for another tick-up in market share.
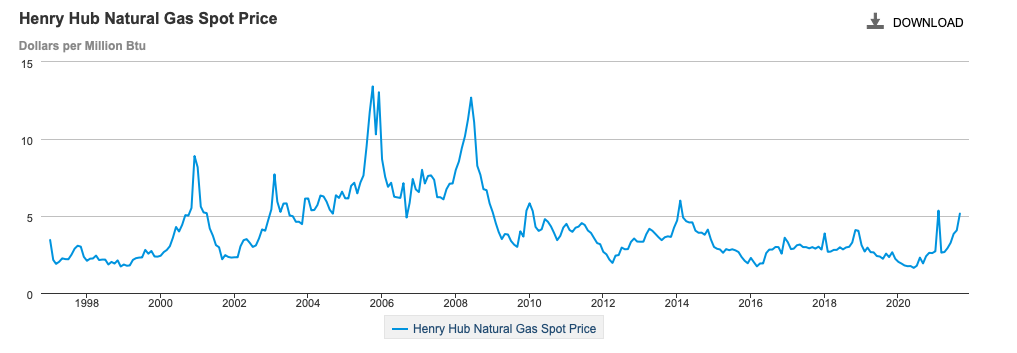
Source: EIA
It is easy to get a lot of renewables, but making them 100% of generation has rapidly diminishing returns. It is why I usually predict 10%-15% of our grid electricity generation being natural gas.
Where Does the Extra Gas Go?
Natural gas power plants are currently the largest source of electricity in the US. Renewable growth should decrease gas demand. Total gas demand in power generation may not fall as fast as its market share if electricity usage grows from the electrification of transport.

Source: EIA
Natural gas exports are what is going wild. The rest of the world needs gas to heat homes, power industrial processes, and generate the marginal kilowatt-hour.

Plus a few more BCF/d to Mexico by pipeline. Source: EIA
There may not even be that much extra gas because a significant portion of supply growth has been a byproduct of oil production. If oil demand slows or starts falling, gas supply in basins like the Permian will as well.
Any gas we don't use is freed up for export to replace coal peaking power plants abroad or prevent people from cutting down trees or mining coal for cooking and warmth. The more the US exports, the more our domestic natural gas price will reflect global prices. Our high natural gas price scenario could happen without a carbon tax.
Assessing US Gas Supply
While the world focused on the COVID-19 pandemic, the US oil and gas industry underwent a monumental change in management practices. Most managers at public companies and many private companies were paid based on gross production. Production volume grew while cash flow was negative. Hot money flowed in from all over the world to get a piece of the shale revolution. Investors finally tired of this and changed pay to incentivize return on invested capital. The industry returned to positive cash flow.
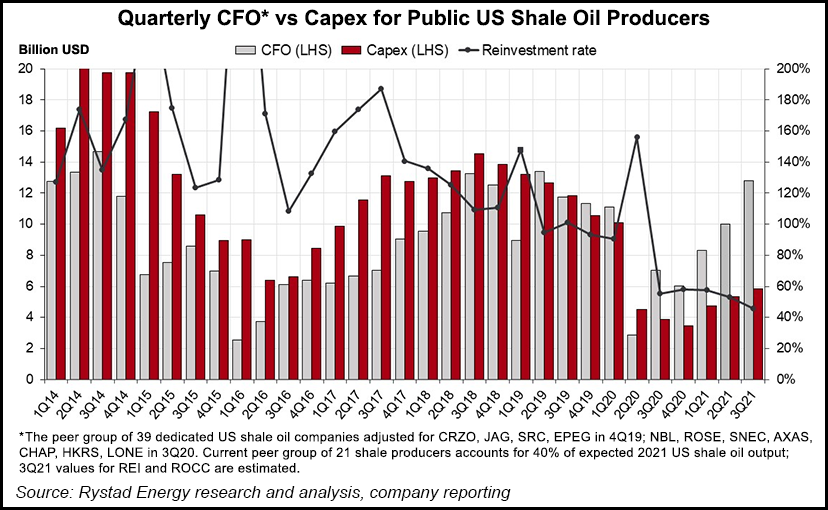
Source: Rystad Energy
Old wells decline in production over time, and shale wells decline even faster. Maintaining production requires constant drilling. Only the Marcellus/Utica in Appalachia, the Haynesville in N. Louisiana and E. Texas, and the Permian Basin in Texas and New Mexico can produce new gas under the new regime at current prices.
Gas supply in the Permian Basin is almost solely a byproduct of oil production and does not respond to gas market signals.
Appalachia is pipeline constrained. Processing and moving gas is the majority of production costs. The best acreage has roughly ten years of drilling locations left at current production rates. It takes 8-10 years to pay off a new pipeline. The old incentive model encouraged new pipeline capacity and volume growth. The new one favors holding production steady and generating more free cash flow as previous pipeline investments depreciate. Building new pipelines enrich pipeline investors instead of E+P investors.
The Haynesville is now setting the price on the supply side and is effectively the only play that can balance supply with demand at prices under $5/MCF. Bringing the Eagleford, Barnett, Arkoma, Powder River, DJ, Fayetteville, and Anadarko Basins back into the fold while generating free cash flow will probably take ~$5-$8/MCF. Drilling productivity improvements are tailing off as the technology is more mature. More plays will crossover the point where declining acreage quality overwhelms productivity growth in the next decade. It is safe to say that natural gas will not maintain primacy as a baseload generation source at greater than $5/MCF.
Without new technology, an era of low gas prices will end. And new technology requires higher prices to incentivize development.
International Gas Supply
The United States has always exported oil and gas technology and expertise. They call Saudi Arabia's national oil company Arabian-American Oil Company (ARAMCO) because it was American companies that found and developed the reserves before their stakes were nationalized.
The United States is one of the only places with private mineral rights. Small mineral holders allow new, innovative drillers to lease easier than they would from a central government. They also form a large constituency that encourages the development of mineral resources. The US has a thicker oilfield services sector and more competition in drilling than anywhere else in the world.
Developing a non-US oil field requires dramatically higher reservoir quality to justify the extra costs. Massive companies with help from expensive, blue-chip service companies dominate the development of non-US reserves. Most prospects that pencil out are megaprojects that can take decades to develop.
Independents drilling tens of thousands of wells drive the US shale revolution in a process that looks more like manufacturing than wildcatting. The complications from government mineral ownership and thin competition raise the cost beyond the economic limit and stifle growth elsewhere. Argentina, Poland, the UK, and the UAE are still net gas importers! At least Argentina is trying. Maybe one or two countries will break out.
Country Spotlight: Saudi Arabia
Saudi Arabia is the sixth-largest natural gas producer and has ample gas reserves. Yet, it cannot produce enough gas to power its own electricity sector and burns around half a million barrels a day of expensive crude oil to produce electricity.
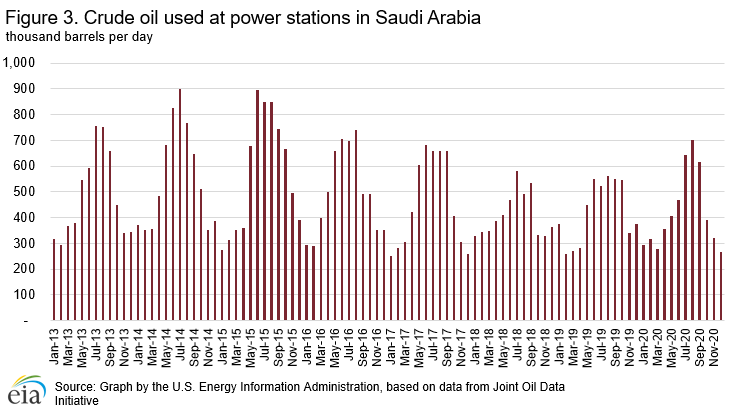
Despite attempts starting in 1975 to increase gas production to reduce oil burn for electricity generation, ARAMCO and its contractors can't keep up with demand. The fuel alone for burning oil amounts to ~$120/MWh at $60 per barrel oil prices. It is a tragedy.
Saudi Arabia is sunny, and its oil-fired generation mostly meets air conditioning demand. New solar would be somewhere around $30/MWh. Roughly 50 GW of solar - with little need for storage - would almost take oil burn to zero. It is not an impossible quantity of solar. China has installed 50 GW of solar in a year multiple times. Saudi Arabia could end its oil-burning within a year or two, especially if they do something rash like an uncapped solar feed-in-tariff paying $50/MWh. Installing panels is a lot easier than drilling gas wells, after all. Yet solar provides less than 0.5% of its electricity.
The Saudi situation is the norm. Most places can't get gas out of the ground even if their lives depended on it. And their energy policies are in shambles. The world needs American exports.
Handling Direct Carbon Emissions
If we are stuck with gas, can it be cleaner?
One Weird Trick
The auto industry is gearing up to put two ~75 kWh (or more!) electric cars in every garage.
Even the NREL zero-carbon scenario is only around 20 kWh per person. The low solar cost, 20% nuclear scenario to get us over 80% zero-carbon only requires 5 kWh per person. Ignoring the capability of cars sending energy into the grid, simply adjusting charging time based on grid conditions can massively increase renewables penetration.
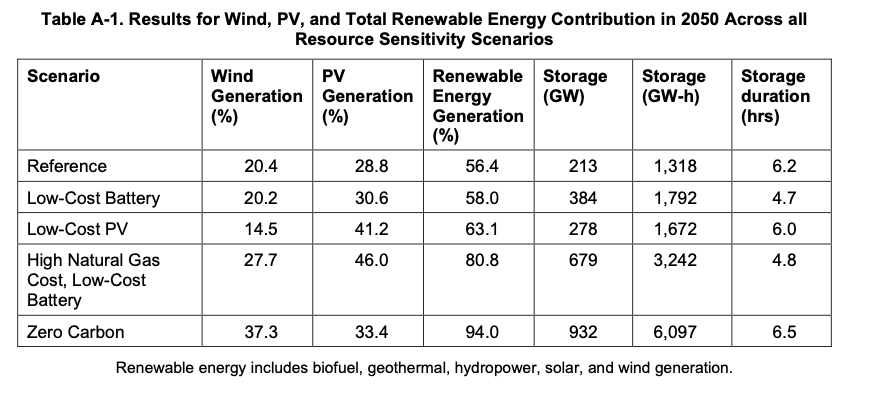
Cars use a lot more batteries than grid storage. Source: NREL
As I discussed in my grid stability post, getting people to change their thermostat based on variable pricing is less impactful than hoped. But electric cars can go days without charging based on typical driving patterns. If drivers charge during times of excess supply, it raises revenue for inflexible producers and justifies more capacity. That capacity meets demand when non-transportation usage is high.
If you want to lower future natural gas usage, incentivizing driver charge behavior might be the highest leverage policy to do that. The opposite is true, as well. Adding a lot of demand that doesn't respond to market conditions will inject unpleasant variation into the grid and increase demand for gas peaking. While we may see some vehicle-to-grid where infrastructure is up to snuff, batteries only have so many cycles on them. It will take more money to encourage drivers to shorten the life of their vehicles in service of the grid. Focusing on when vehicles charge is easy money.
Direct Air Capture and Carbon Taxes
Putting carbon capture equipment on a power plant that runs 10% of the time or on a residential furnace is unrealistic.
The impact of carbon taxes on demand quickly diminishes as demand becomes more inelastic. A carbon tax would push gas generation further out of baseload towards peaking duty. $100/ton of carbon dioxide adds ~$50/MWh to a combined-cycle natural gas plant and ~$100/MWh to a simple cycle one. Markets will pay this price because natural gas peaking plants are one of the last lines of defense. If DAC becomes cheaper than the carbon tax, plant owners will capture the carbon instead of paying the tax.
It makes sense for low utilization, inelastic emitters to buy capture capacity from specialized producers running at higher utilization.
Carbon Tax Politics
Europe, California, the Northeast US, and China already have explicit carbon pricing in some sectors.
Other taxes are more complicated. Are excise and severance taxes on fossil fuels carbon taxes? While these other taxes may increase the cost of fossil fuels, they do not incentivize carbon capture.
The popularity of carbon taxes seems to follow the will to generally tax energy. Europe had sky-high fuel taxes long before cap and trade. California and the Northeast US regularly enact policies that increase local energy prices. China has high excise taxes on (mostly imported) liquid fuel. The carbon tax on domestic coal is small.
Carbon taxes will be more acceptable if the median American in 2040 is driving an electric vehicle and coal power plants are a thing of the past. It is easy to imagine kludges like every household getting carbon tax-free heating fuel below a certain usage amount. Sectors that are difficult to decarbonize might get picked off for specific carbon taxes.
Opinions on carbon taxes can shift.
Emissions Free Power Plants
NetPower's Allam Cycle natural gas power plant is an interesting new climate technology. It burns methane in pure oxygen, meaning exhaust is only water and carbon dioxide. The plant captures the CO2 easily. There are no SOx, NOx, or particulate emissions. NetPower has been running a 25 MW demonstration plant in Texas for several years and plans to start construction on the first commercial plants shortly.
Techno-economic analysis generally agrees that Allam Cycle plants are more expensive than combined cycle plants but cheaper than adding carbon capture on existing plants. The Allam Cycle design replaces both the gas turbine and the steam turbine with one supercritical CO2 turbine, has a smaller footprint, and uses less water. It adds the expense of separating oxygen from the air and uses a complex heat exchanger to recover heat from the turbine exhaust.
Economic success requires either a price on carbon or a market to sell CO2. New plants are unlikely to replace existing peakers in the US anytime soon, but they could find niches in co-locating with CO2 using industries.
The best hope for almost every new non-solar, wind, or lithium battery technology to grow are markets like Northern Europe, where sunlight is hard to come by, and the government taxes carbon dioxide emissions. The Allam Cycle could give advanced geothermal or new nuclear good competition even at high natural gas prices. Its extra flexibility and lower CAPEX are a big plus in high wind penetration grids like Northern Europe has.
Synthetic Fuel
Synthetic methane produced from carbon-free energy sources provides a long-term solution to seasonal storage and high-density energy needs like peaking and building heat.
A New Engineering Paradigm
In my heating post, I went over many possible technological pathways to synthetic fuels. Traditionally, fuel price spikes have spurred innovation in oil and gas technology that increases supply and lowers prices. At some point, a price spike might spur innovation in synthetic fuels instead.
Recently we have seen startups like TerraForm Industries and SpaceX aim to dramatically lower the cost of synthetic fuels and make methane from hydrogen and CO2.
Traditionally, chemical engineers have had expensive, always available energy in natural gas and oil to use. The most economical plant designs favor higher capital expenses to increase energy efficiency and running plants 24/7 at a massive scale to make the economics work. Several generations of engineers had these heuristics beaten into their heads.
New technologies give us cheap energy that is variable. The optimal design paradigm changes from being efficient and capital intensive to being inefficient and simple. Current electrolyzers to make hydrogen from water are efficient. They are also incredibly complex and expensive. The other end of the paradigm is an electrolyzer made up of two sharpened No. 2 pencils stuck in a jar of salt water. Much of engineering analysis on synthetic fuels assumes the old paradigm.
Synthetic Natural Gas
Hydrogen + Carbon Dioxide + Energy -> Methane + Water.
As solar costs fall, a cheap electrolyzer producing hydrogen can utilize the electricity only running part of the day. Co-location with solar also has other benefits. Electrolyzers use direct current electricity, which is what solar panels produce. And the voltages are similar. The electricity cost of powering electrolyzers with on-site solar is less than what it costs to go into a grid. Batteries see a similar effect where co-location reduces capital cost by 25%. Developers can avoid pesky problems like grid interconnection agreements.
Wind-dominated grids work differently. At high penetrations, there is often over-generation that congests the transmission network and forces curtailment. These events can go on for days at a time, much longer than batteries can economically store power. Wind turbines produce AC power, so no power electronics savings. But there is near-free electricity available. If competition for stranded electricity increases from players like iron-air batteries, it will raise the return to overbuilding wind and expand electricity supply.
Feedstock CO2 is captured from the air or collected from industrial sources. An x-factor for carbon-based synthetic fuels is that carbon dioxide reacts with lime and hydroxides, making concentration easier than CO2's 400 ppm atmospheric concentration seems. Carbon Engineering, assuming $80/MWh electricity costs and Western Canada labor costs, estimated their system delivers CO2 from the air at $100-$200 per ton. Designs taking advantage of cheap electricity could cost a lot less.
Making methane this way starts at around 30% efficiency, meaning each MCF of gas requires ~1 MWh. Even with ultra-low solar costs, gas might still cost $10/MCF. Getting under $10/MCF would be competitive in most of the world besides the US, especially with carbon pricing. A $100 per ton tax on CO2 adds $5.50 to an MCF. Within the US, places like California and New England have high gas prices supportive of synthetic fuels. An advantage of low-CAPEX solutions is that they have to be simple and use highly available materials by default. If there is a cost crossover, growth is rapid. Unlike natural gas wells, the best acreage doesn't run out.
Turning sunlight and other inflexible energy sources into drop-in fuels is a strategy that has promise.
Ammonia and the New Engineering Paradigm
Most US ammonia producers use natural gas as a feedstock to make ammonia in multi-billion-dollar Haber-Bosch facilities. These plants are only getting bigger. The most prolific plant designer, Kellogg, is touting 6000 tons/day trains, up from 1500-3000 tons/day. "Green" ammonia fuel advocates hope to go toe-to-toe with traditional facilities using hydrogen made from electrolysis instead of getting hydrogen from natural gas.
Winning is a challenge because of scale and the low cost of feedstock. $5/MCF equates to $17/MWh. The electrolysis route requires more energy using typical plant configurations. There is very little chance of getting sub-$15/MWh electricity 24 hours a day with any emerging technology. Plants must run at lower utilization to have competitive electricity prices or store hydrogen. A traditional Haber-Bosch plant might take 30 hours just safely start or shut down, making the storage route more likely.
A company named Nitricity uses the new paradigm to manufacture fertilizer. They produce NOx by using electricity to crack nitrogen and oxygen molecules. Then they bubble NOx through water, which makes dilute nitric acid. The nitric acid can go straight on the field in alkaline soil. Farmers mix it with potassium hydroxide or limestone to balance the pH for neutral or acidic soils. Solar panels, without storage or grid connections, power the setup.
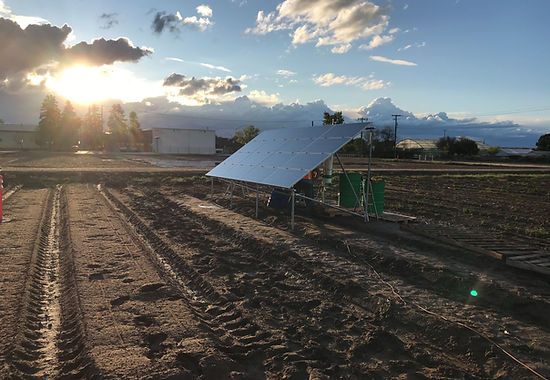
The pilot fits under some solar panels and is only a few components. Source: Nitricity
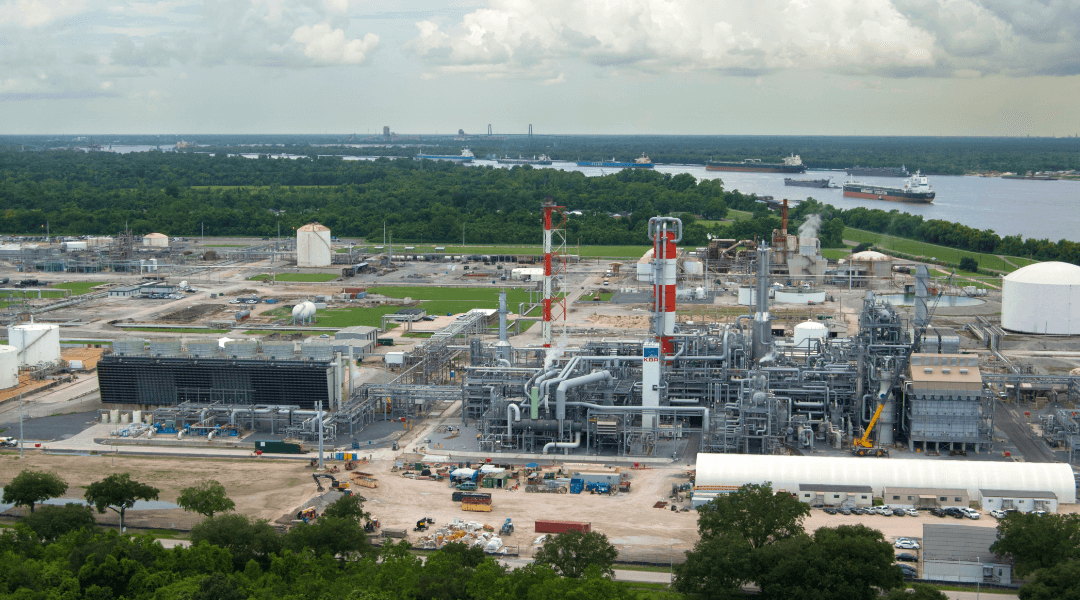
Complexity of Haber Bosch design ammonia plants is so high that massive scale is a requirement. Source: KBR
The current process is an energy hog, using 70 MWh/ton of nitrogen compared to 8-9 MWh/ton of ammonia for Haber-Bosch. Several items balance the energy consumption:
-
Nitrogen Efficiency
The Nitricity system feeds small amounts of fertilizer into the irrigation system regularly instead of applying fertilizer in batches. Depending on conditions, a field might only require one-third of the nitrogen as with batch applications.
-
Shipping Costs
Transportation and distribution costs loom large for cheap commodities like fertilizer. The farmer's price might be 2-3x the price at the plant. Smaller installations on-farm or nearby minimize supply chain costs.
-
Lower CAPEX Potential
One ton per year of capacity with a new Haber Bosch plant costs around $1500. Adding urea, nitric acid, and ammonium nitrate plants increases the total. After building a few test systems at ~1/100,000 scale of H-B plants, Nitricity also costs around $1500 to add a ton per year (running at 100% capacity). There is room for CAPEX to improve dramatically through scale, iteration, and increasing efficiency.
-
Reduced Labor Costs
Instead of driving a tractor to apply the fertilizer, it integrates into automated irrigation systems.
The upshot is that Nitricity is price competitive even at prototype scale where farms are sunny, far from fertilizer plants, use irrigation, and have alkaline soil. Improvements in cost and efficiency paired with falling solar panel costs threaten to reduce demand for natural gas to make ammonia - somewhere between 5%-10% of global gas demand. Fertilizer is only one example of the possible engineering paradigm shift.
24/7 Process Heat
Natural gas dominates the market for process heat in factories and chemical plants. Renewables under $15/MWh start competing on a direct energy basis with natural gas. The startup Rondo wants to turn cheap, variable electricity into on-demand process heat. The plan is to heat bricks to 2200 F with electric resistance heating. Resistance heaters and bricks are cheap. The facility can remove heat from the bricks as needed. Round trip efficiency can be over 85% - much higher than making hydrogen or synthetic fuels.
Gas is more vulnerable to renewables in applications with regular daily demand than more sporadic use cases like building heat.
Solving Methane Emissions
Many environmental attacks on gas focus on methane leaks. While methane's longevity in the atmosphere is short compared to carbon dioxide, its warming potential per ton is higher. Environmentalists are concerned about leaks from wells, pipelines, and distribution systems.
It turns out that leaks themselves are usually inexpensive to fix, but they are expensive to find. It is hard to know you even have one. A sleepy well might cost as little as $400 in labor per month to operate. Checking for methane leaks requires an expensive tech to haul a $25,000 thermal camera to each remote site. Checking for pipeline leaks requires workers on four-wheelers to trace lines across fields and fences with expensive gas sniffers. Fixing a dump valve or small hole can cost less than $100, but you might spend thousands of dollars to find out you have a leak in the first place.
Low-cost satellites and launch providers change the math. A charity plans to launch MethaneSAT in the fall of 2022 on a SpaceX rocket. The satellite will find point source emissions in better detail than any existing satellite by detecting methane at parts per billion levels with sub-100-meter resolution. The charity estimates the entire project costs $88 million. Data will be public, and the satellite can image most of the globe every few days. There are almost two million oil and gas wells in the US alone. Not to mention the pipelines, landfills, and distribution systems the satellite can cover as well. The cost of leak detection can drop orders of magnitude.
Data allows efficient taxing or repair of leaks. Cities can determine where their gas systems need updating. Satellites move methane emissions from an existential bludgeoning to a straightforward problem.
The Long Game
Exports, tightening supply, carbon pricing, and increasing renewables penetration will push gas more into seasonal and peaking duties over the coming decades. A carbon tax would increase gas consumption by encouraging coal-to-gas switching in the short term. After the coal is gone, it will raise the price in applications where gas has a strong comparative advantage until carbon capture is less costly.
Over time the gas system will become cleaner as methane leaks diminish, new power plants replace the old, synthetic fuel production grows, and DAC options become cheaper than carbon pricing. The politics of carbon pricing will become more palatable once fossil fuels like coal and oil are not setting the everyday marginal price of energy for voters. While drilling might become less common, there will still be a rump of existing wells that produce gas at low prices for decades to come.
The chemical industry uses electricity, gas, and oil based on relative pricing and comparative advantage. Pricier gas, falling electricity prices, transport electrification, and cheap electrochemistry-based technologies could shift the balance towards electricity and oil.
Because new infrastructure is expensive, there is almost certainly going to be some amount of gas generation that remains the cheapest generation even with massive cost declines in other technologies. In 50 years, when our current set of gas power plants starts to reach end-of-life, there might be a surplus of depreciated battery storage that has more value running 20 cycles a year as peak capacity than scrap. Cheap, distributed electricity generation that avoids grid costs is the only technology that could upend this dynamic outside of massive political intervention.
Many climate activists support electrification in anticipation of a cleaner electricity grid. We should begin to anticipate and work towards a better gas system.
-
NREL Storage Futures Study
-
FERC Report on Natural Gas Storage
-
Natural Gas Power Plant Capacity - EIA
-
EIA Electricity Demand Stats
Natural Gas is a Fuel of the Future
2022 February 23 Twitter Substack See all postsInertia and new technology make it hard and undesirable to root out.
Why Gas is Useful
Efficient, Cheap Power Plants
Gas power plants are cheap.
Source: Lazard
Why are gas plants so cheap? They have less equipment. A gas turbine takes in compressed gas and air, burns it, spits out the exhaust, and turns a generator. Modern turbines are as efficient as coal or nuclear plants and a fraction of the size of hulking steam turbines. They are 3x as efficient as the average geothermal facility.
Coal, nuclear, and geothermal plants utilize more complex thermodynamic cycles. They have a boiler, a steam turbine, a generator, a condenser, a boiler feed water pump, more cooling towers, and a water purification system for boiler feed water. Coal plants contend with solids handling, and nuclear plants have complex reactors.
Combined cycle natural gas power plants use hot exhaust from the turbine to make steam like traditional thermal plants. About 2/3 of plant output comes from the gas turbine and 1/3 from the steam turbine. Total efficiency can be over 60% with less equipment and labor than boiler-style thermal plants.
Cheap Storage
Most natural gas storage is in depleted reservoirs. They fill up in the summer when gas demand is low and empty in the winter when gas demand is high.
Building a depleted reservoir gas storage facility costs about $6 million per billion cubic feet of gas. That equates to $0.02/kWh. A grid storage lithium-ion battery currently costs $250-$300/kWh.
The marginal cost of storing gas is determined by renting space in the storage facility and compressing it into the reservoir, usually ~$0.50/MCF for a season. Spot natural gas prices have been between $1.50 and $6 over the last decade. Efficiency determines the marginal cycle cost for a battery. Most lithium-ion battery systems are 90%+ efficient. Tesla's Powerwall has a 92.5% round trip efficiency.
Reservoir permeability limits gas injection/discharge rates to emptying once per season. Salt dome storage facilities are an exception that can cycle faster but have higher construction costs. Batteries can cycle within a few hours.
Batteries have an advantage in short-term storage, while natural gas storage is much better for long-duration storage.
Better Than X
Hydrogen
Why not store hydrogen instead of natural gas?
Hydrogen embrittles metal that pipelines and storage facility wells are made of, limiting usage in existing infrastructure.
Methane (the primary molecule in natural gas) has three times the energy per volume as hydrogen. A switch to hydrogen would mean we'd need three times more pipeline, compressor, and gas storage capacity.
Hydrogen is more expensive to store because of poor volumetric energy density, and it needs all new infrastructure. If we see widespread hydrogen storage, it will likely be local and only for industrial and electricity use. Building new interstate pipelines is increasingly difficult. As we've seen with electricity transmission, critics do not make an exception for "green" projects. I will remain skeptical.
Better Than Air
Compressed air storage also uses caverns and reservoirs as cheap, long-term storage. Compressed methane is ~80x more energy-dense than compressed air. Facilities need new turbines and grid connections, unlike natural gas.
Ammonia
Ammonia is easy to liquefy, so it has good volumetric energy density. Proponents favor applications like marine fuel and gas turbines.
The downsides are that it is poisonous, burns slow, releases tons of NOx when burned, and is less dense than regular ship fuel. The toxic aspect eliminates its use in residential applications. The combustion characteristics mean turbines need larger combustion chambers and more emissions control than natural gas turbines. And the lower volumetric density means it is vulnerable to drop-in synthetic liquid fuels in maritime applications.
Pumped Hydro and Other Cats and Dogs
Pumped hydro costs a thousand times more per unit of energy than natural gas storage. All the other random pet technologies are expensive, too. Storing energy in hydrocarbons is laughably cheap. Competing technologies tend to be awkward tweeners. They can't compete against batteries in short-term storage or against gas in longer-duration storage.
Electric Home Heating
A previous post covers why it is best to let markets sort out heating fuel rather than mandating electrification. Heating can require massive amounts of energy in a short time. Natural gas handles this volatility better than electric grids.
If utilities charge households correctly for electricity use, electric heating looks less economical in many places. Residential customers usually only pay for the kilowatt-hours they use. Costs like distribution, transmission, and building power plants are all rolled in. It is much cheaper for utilities to serve a customer that uses electricity consistently than one that uses the same total amount at sporadic rates. Large power users pay for each kilowatt-hour and a "demand" fee based on their peak usage. Demand charges might be half a big user's bill. Non-electric heat customers subsidize customers with electric heating who see power spikes on a few cold days a year. Grid stress increases because the utility didn't collect demand charges to build out low utilization power plants and transmission lines.
The dynamic can flip in hot places where cold snaps are non-existent. The gas heat home uses electricity at a high rate in the summer with low usage in winter. The electric heat home raises the utilization of the system without requiring new investment.
When markets send the correct signals, they maximize reliability and minimize costs.
Dispatchable
Zero carbon resources like geothermal, nuclear, solar, nuclear, and wind have mostly fixed costs. Upfront costs are high, and variable cost is low. Coal, natural gas, and hydro (usually) are dispatchable. Gas and coal have lower fixed costs and higher marginal (fuel) costs, while limited hydropower reservoir levels add opportunity costs.
Geothermal and nuclear may technically be dispatchable, but it is expensive. Running in load-following mode decreases revenue and adds costs by increasing labor and maintenance. Running a nuclear or geothermal plant at 50% capacity more than doubles the cost of electricity it produces. Like solar, wind, and batteries, their CAPEX must fall even further to increase market share.
The average peaker only runs at about 11% of its full utilization. Replacing peakers with 24/7 power sources like nuclear and geothermal would increase their cost per megawatt-hour by roughly 10x. The fact that natural gas peakers already exist makes the economics for competitors even worse. Adding batteries and renewables can extend the life of existing gas peaking plants because instead of starting every evening to run for an hour, they run in longer spurts when demand and prices are high. Turbine maintenance and lifetime are both impacted by cycles run.
While we may imagine our electricity use to be steady, variance is high. There is only so much room for nuclear or geothermal before gas turbines, batteries, or import/export capability are much cheaper than continuing to add nuclear or geothermal capacity. It ends up that sources like solar and nuclear are closer substitutes than commonly assumed. Only grids with high hydro penetration can do without gas or coal at a reasonable cost.
Demand changes a lot even in short periods
Gas does the hard work of matching supply and demand. Source: EIA
There are ideas like powering existing coal plant steam turbines with hot, dry rock geothermal steam that may have a chance to nose in. The challenge is still CAPEX. Even with the bluest sky optimistic assumptions, it would take $10/MCF and $20/MCF gas prices to put a retrofit facility in the green against existing simple cycle turbines and combined-cycle plants at 10% capacity factors. There might not be any coal plants to retrofit once the technology and a sufficient carbon tax exist. Or competing technologies like synthetic natural gas or cheap carbon capture could swoop in under $10/MCF. It may be a better option for places like Asia with higher gas prices, fewer existing gas plants, and a surfeit of coal plants.
Source: Lazard
Natural Gas Power Plant Inventory
Natural gas power plants make up 43% of US electricity generation capacity. About half of that capacity is relatively new combined-cycle plants. Gas turbines and a raft of gas-fired steam turbines make up the rest.
Source: EIA
Combined cycle plants shoulder most of the burden matching daily and seasonal electricity demand patterns. The steam and gas turbine peaker plants see most of their use on summer afternoons and evenings. Gas-fired steam turbine plants are usually more efficient than older gas turbines which can be as low as 20% efficiency. New turbines can be as high as 35% efficient. It is hard to kill depreciated plants in low-utilization applications.
Gas power plants compete directly with coal power plants in providing baseload power and meeting peaking/seasonal demand. Coal-to-Gas switching is one of the relief valves for balancing gas supply and demand. When prices are high, coal burns more, and when prices are low, gas burn increases. Utilities are closing most coal power plants because they are older, have higher fixed costs, and environmental compliance is more expensive.
As renewables increase market share, coal will decrease while combined cycle plants move towards seasonal and peaking duty. These plants can survive long past their estimated service date because of low fixed costs. Solar and batteries can meet typical afternoon/evening peaks cost-effectively. Gas power plants might run more of the day when sun or wind resources are less available instead of running for a few hours on hot afternoons. Natural gas prices might become more volatile, but fuel is a smaller share of costs in applications like home heating and peak electricity generation.
It is funny when pundits criticize renewables for needing backup generation when they already pay for such a system every month on their electric bill.
How Much Gas Do We Need?
The National Renewable Energy Laboratory recently released a study showing market share based on various scenarios.
Cheap Solar = $15/MWh, Cheap Batteries = $80/kWh, Baseline Gas = $3.50/MCF, Expensive Gas = $6.50/MCF Source: NREL
Even with conservative assumptions about cost declines and assuming natural gas prices at $3.50/MCF, non-nuclear renewables will make up a large share of our grid by 2050. Every solar panel and battery added to the grid sees lower utilization, requiring cost decreases for higher penetration. Assuming 20% nuclear generation market share, 83% of our electricity would be zero-carbon even with cheap gas.
Adding low utilization renewables and batteries become more competitive if natural gas prices are high. High is ~$6.50/MCF in this example. That price is incredibly low for Europe and Asia and is not historically high for the US. Grid battery storage doubles to meet the new demand in this scenario. Using zero gas requires doubling storage again and a massive buildout of wind and long-distance transmission (yikes!) for another tick-up in market share.
Source: EIA
It is easy to get a lot of renewables, but making them 100% of generation has rapidly diminishing returns. It is why I usually predict 10%-15% of our grid electricity generation being natural gas.
Where Does the Extra Gas Go?
Natural gas power plants are currently the largest source of electricity in the US. Renewable growth should decrease gas demand. Total gas demand in power generation may not fall as fast as its market share if electricity usage grows from the electrification of transport.
Source: EIA
Natural gas exports are what is going wild. The rest of the world needs gas to heat homes, power industrial processes, and generate the marginal kilowatt-hour.
Plus a few more BCF/d to Mexico by pipeline. Source: EIA
There may not even be that much extra gas because a significant portion of supply growth has been a byproduct of oil production. If oil demand slows or starts falling, gas supply in basins like the Permian will as well.
Any gas we don't use is freed up for export to replace coal peaking power plants abroad or prevent people from cutting down trees or mining coal for cooking and warmth. The more the US exports, the more our domestic natural gas price will reflect global prices. Our high natural gas price scenario could happen without a carbon tax.
Assessing US Gas Supply
While the world focused on the COVID-19 pandemic, the US oil and gas industry underwent a monumental change in management practices. Most managers at public companies and many private companies were paid based on gross production. Production volume grew while cash flow was negative. Hot money flowed in from all over the world to get a piece of the shale revolution. Investors finally tired of this and changed pay to incentivize return on invested capital. The industry returned to positive cash flow.
Source: Rystad Energy
Old wells decline in production over time, and shale wells decline even faster. Maintaining production requires constant drilling. Only the Marcellus/Utica in Appalachia, the Haynesville in N. Louisiana and E. Texas, and the Permian Basin in Texas and New Mexico can produce new gas under the new regime at current prices.
Gas supply in the Permian Basin is almost solely a byproduct of oil production and does not respond to gas market signals.
Appalachia is pipeline constrained. Processing and moving gas is the majority of production costs. The best acreage has roughly ten years of drilling locations left at current production rates. It takes 8-10 years to pay off a new pipeline. The old incentive model encouraged new pipeline capacity and volume growth. The new one favors holding production steady and generating more free cash flow as previous pipeline investments depreciate. Building new pipelines enrich pipeline investors instead of E+P investors.
The Haynesville is now setting the price on the supply side and is effectively the only play that can balance supply with demand at prices under $5/MCF. Bringing the Eagleford, Barnett, Arkoma, Powder River, DJ, Fayetteville, and Anadarko Basins back into the fold while generating free cash flow will probably take ~$5-$8/MCF. Drilling productivity improvements are tailing off as the technology is more mature. More plays will crossover the point where declining acreage quality overwhelms productivity growth in the next decade. It is safe to say that natural gas will not maintain primacy as a baseload generation source at greater than $5/MCF.
Without new technology, an era of low gas prices will end. And new technology requires higher prices to incentivize development.
International Gas Supply
The United States has always exported oil and gas technology and expertise. They call Saudi Arabia's national oil company Arabian-American Oil Company (ARAMCO) because it was American companies that found and developed the reserves before their stakes were nationalized.
The United States is one of the only places with private mineral rights. Small mineral holders allow new, innovative drillers to lease easier than they would from a central government. They also form a large constituency that encourages the development of mineral resources. The US has a thicker oilfield services sector and more competition in drilling than anywhere else in the world.
Developing a non-US oil field requires dramatically higher reservoir quality to justify the extra costs. Massive companies with help from expensive, blue-chip service companies dominate the development of non-US reserves. Most prospects that pencil out are megaprojects that can take decades to develop.
Independents drilling tens of thousands of wells drive the US shale revolution in a process that looks more like manufacturing than wildcatting. The complications from government mineral ownership and thin competition raise the cost beyond the economic limit and stifle growth elsewhere. Argentina, Poland, the UK, and the UAE are still net gas importers! At least Argentina is trying. Maybe one or two countries will break out.
Country Spotlight: Saudi Arabia
Saudi Arabia is the sixth-largest natural gas producer and has ample gas reserves. Yet, it cannot produce enough gas to power its own electricity sector and burns around half a million barrels a day of expensive crude oil to produce electricity.
Despite attempts starting in 1975 to increase gas production to reduce oil burn for electricity generation, ARAMCO and its contractors can't keep up with demand. The fuel alone for burning oil amounts to ~$120/MWh at $60 per barrel oil prices. It is a tragedy.
Saudi Arabia is sunny, and its oil-fired generation mostly meets air conditioning demand. New solar would be somewhere around $30/MWh. Roughly 50 GW of solar - with little need for storage - would almost take oil burn to zero. It is not an impossible quantity of solar. China has installed 50 GW of solar in a year multiple times. Saudi Arabia could end its oil-burning within a year or two, especially if they do something rash like an uncapped solar feed-in-tariff paying $50/MWh. Installing panels is a lot easier than drilling gas wells, after all. Yet solar provides less than 0.5% of its electricity.
The Saudi situation is the norm. Most places can't get gas out of the ground even if their lives depended on it. And their energy policies are in shambles. The world needs American exports.
Handling Direct Carbon Emissions
If we are stuck with gas, can it be cleaner?
One Weird Trick
The auto industry is gearing up to put two ~75 kWh (or more!) electric cars in every garage.
Even the NREL zero-carbon scenario is only around 20 kWh per person. The low solar cost, 20% nuclear scenario to get us over 80% zero-carbon only requires 5 kWh per person. Ignoring the capability of cars sending energy into the grid, simply adjusting charging time based on grid conditions can massively increase renewables penetration.
Cars use a lot more batteries than grid storage. Source: NREL
As I discussed in my grid stability post, getting people to change their thermostat based on variable pricing is less impactful than hoped. But electric cars can go days without charging based on typical driving patterns. If drivers charge during times of excess supply, it raises revenue for inflexible producers and justifies more capacity. That capacity meets demand when non-transportation usage is high.
If you want to lower future natural gas usage, incentivizing driver charge behavior might be the highest leverage policy to do that. The opposite is true, as well. Adding a lot of demand that doesn't respond to market conditions will inject unpleasant variation into the grid and increase demand for gas peaking. While we may see some vehicle-to-grid where infrastructure is up to snuff, batteries only have so many cycles on them. It will take more money to encourage drivers to shorten the life of their vehicles in service of the grid. Focusing on when vehicles charge is easy money.
Direct Air Capture and Carbon Taxes
Putting carbon capture equipment on a power plant that runs 10% of the time or on a residential furnace is unrealistic.
The impact of carbon taxes on demand quickly diminishes as demand becomes more inelastic. A carbon tax would push gas generation further out of baseload towards peaking duty. $100/ton of carbon dioxide adds ~$50/MWh to a combined-cycle natural gas plant and ~$100/MWh to a simple cycle one. Markets will pay this price because natural gas peaking plants are one of the last lines of defense. If DAC becomes cheaper than the carbon tax, plant owners will capture the carbon instead of paying the tax.
It makes sense for low utilization, inelastic emitters to buy capture capacity from specialized producers running at higher utilization.
Carbon Tax Politics
Europe, California, the Northeast US, and China already have explicit carbon pricing in some sectors.
Other taxes are more complicated. Are excise and severance taxes on fossil fuels carbon taxes? While these other taxes may increase the cost of fossil fuels, they do not incentivize carbon capture.
The popularity of carbon taxes seems to follow the will to generally tax energy. Europe had sky-high fuel taxes long before cap and trade. California and the Northeast US regularly enact policies that increase local energy prices. China has high excise taxes on (mostly imported) liquid fuel. The carbon tax on domestic coal is small.
Carbon taxes will be more acceptable if the median American in 2040 is driving an electric vehicle and coal power plants are a thing of the past. It is easy to imagine kludges like every household getting carbon tax-free heating fuel below a certain usage amount. Sectors that are difficult to decarbonize might get picked off for specific carbon taxes.
Opinions on carbon taxes can shift.
Emissions Free Power Plants
NetPower's Allam Cycle natural gas power plant is an interesting new climate technology. It burns methane in pure oxygen, meaning exhaust is only water and carbon dioxide. The plant captures the CO2 easily. There are no SOx, NOx, or particulate emissions. NetPower has been running a 25 MW demonstration plant in Texas for several years and plans to start construction on the first commercial plants shortly.
Techno-economic analysis generally agrees that Allam Cycle plants are more expensive than combined cycle plants but cheaper than adding carbon capture on existing plants. The Allam Cycle design replaces both the gas turbine and the steam turbine with one supercritical CO2 turbine, has a smaller footprint, and uses less water. It adds the expense of separating oxygen from the air and uses a complex heat exchanger to recover heat from the turbine exhaust.
Economic success requires either a price on carbon or a market to sell CO2. New plants are unlikely to replace existing peakers in the US anytime soon, but they could find niches in co-locating with CO2 using industries.
The best hope for almost every new non-solar, wind, or lithium battery technology to grow are markets like Northern Europe, where sunlight is hard to come by, and the government taxes carbon dioxide emissions. The Allam Cycle could give advanced geothermal or new nuclear good competition even at high natural gas prices. Its extra flexibility and lower CAPEX are a big plus in high wind penetration grids like Northern Europe has.
Synthetic Fuel
Synthetic methane produced from carbon-free energy sources provides a long-term solution to seasonal storage and high-density energy needs like peaking and building heat.
A New Engineering Paradigm
In my heating post, I went over many possible technological pathways to synthetic fuels. Traditionally, fuel price spikes have spurred innovation in oil and gas technology that increases supply and lowers prices. At some point, a price spike might spur innovation in synthetic fuels instead.
Recently we have seen startups like TerraForm Industries and SpaceX aim to dramatically lower the cost of synthetic fuels and make methane from hydrogen and CO2.
Traditionally, chemical engineers have had expensive, always available energy in natural gas and oil to use. The most economical plant designs favor higher capital expenses to increase energy efficiency and running plants 24/7 at a massive scale to make the economics work. Several generations of engineers had these heuristics beaten into their heads.
New technologies give us cheap energy that is variable. The optimal design paradigm changes from being efficient and capital intensive to being inefficient and simple. Current electrolyzers to make hydrogen from water are efficient. They are also incredibly complex and expensive. The other end of the paradigm is an electrolyzer made up of two sharpened No. 2 pencils stuck in a jar of salt water. Much of engineering analysis on synthetic fuels assumes the old paradigm.
Synthetic Natural Gas
Hydrogen + Carbon Dioxide + Energy -> Methane + Water.
As solar costs fall, a cheap electrolyzer producing hydrogen can utilize the electricity only running part of the day. Co-location with solar also has other benefits. Electrolyzers use direct current electricity, which is what solar panels produce. And the voltages are similar. The electricity cost of powering electrolyzers with on-site solar is less than what it costs to go into a grid. Batteries see a similar effect where co-location reduces capital cost by 25%. Developers can avoid pesky problems like grid interconnection agreements.
Wind-dominated grids work differently. At high penetrations, there is often over-generation that congests the transmission network and forces curtailment. These events can go on for days at a time, much longer than batteries can economically store power. Wind turbines produce AC power, so no power electronics savings. But there is near-free electricity available. If competition for stranded electricity increases from players like iron-air batteries, it will raise the return to overbuilding wind and expand electricity supply.
Feedstock CO2 is captured from the air or collected from industrial sources. An x-factor for carbon-based synthetic fuels is that carbon dioxide reacts with lime and hydroxides, making concentration easier than CO2's 400 ppm atmospheric concentration seems. Carbon Engineering, assuming $80/MWh electricity costs and Western Canada labor costs, estimated their system delivers CO2 from the air at $100-$200 per ton. Designs taking advantage of cheap electricity could cost a lot less.
Making methane this way starts at around 30% efficiency, meaning each MCF of gas requires ~1 MWh. Even with ultra-low solar costs, gas might still cost $10/MCF. Getting under $10/MCF would be competitive in most of the world besides the US, especially with carbon pricing. A $100 per ton tax on CO2 adds $5.50 to an MCF. Within the US, places like California and New England have high gas prices supportive of synthetic fuels. An advantage of low-CAPEX solutions is that they have to be simple and use highly available materials by default. If there is a cost crossover, growth is rapid. Unlike natural gas wells, the best acreage doesn't run out.
Turning sunlight and other inflexible energy sources into drop-in fuels is a strategy that has promise.
Ammonia and the New Engineering Paradigm
Most US ammonia producers use natural gas as a feedstock to make ammonia in multi-billion-dollar Haber-Bosch facilities. These plants are only getting bigger. The most prolific plant designer, Kellogg, is touting 6000 tons/day trains, up from 1500-3000 tons/day. "Green" ammonia fuel advocates hope to go toe-to-toe with traditional facilities using hydrogen made from electrolysis instead of getting hydrogen from natural gas.
Winning is a challenge because of scale and the low cost of feedstock. $5/MCF equates to $17/MWh. The electrolysis route requires more energy using typical plant configurations. There is very little chance of getting sub-$15/MWh electricity 24 hours a day with any emerging technology. Plants must run at lower utilization to have competitive electricity prices or store hydrogen. A traditional Haber-Bosch plant might take 30 hours just safely start or shut down, making the storage route more likely.
A company named Nitricity uses the new paradigm to manufacture fertilizer. They produce NOx by using electricity to crack nitrogen and oxygen molecules. Then they bubble NOx through water, which makes dilute nitric acid. The nitric acid can go straight on the field in alkaline soil. Farmers mix it with potassium hydroxide or limestone to balance the pH for neutral or acidic soils. Solar panels, without storage or grid connections, power the setup.
The pilot fits under some solar panels and is only a few components. Source: Nitricity
Complexity of Haber Bosch design ammonia plants is so high that massive scale is a requirement. Source: KBR
The current process is an energy hog, using 70 MWh/ton of nitrogen compared to 8-9 MWh/ton of ammonia for Haber-Bosch. Several items balance the energy consumption:
Nitrogen Efficiency
The Nitricity system feeds small amounts of fertilizer into the irrigation system regularly instead of applying fertilizer in batches. Depending on conditions, a field might only require one-third of the nitrogen as with batch applications.
Shipping Costs
Transportation and distribution costs loom large for cheap commodities like fertilizer. The farmer's price might be 2-3x the price at the plant. Smaller installations on-farm or nearby minimize supply chain costs.
Lower CAPEX Potential
One ton per year of capacity with a new Haber Bosch plant costs around $1500. Adding urea, nitric acid, and ammonium nitrate plants increases the total. After building a few test systems at ~1/100,000 scale of H-B plants, Nitricity also costs around $1500 to add a ton per year (running at 100% capacity). There is room for CAPEX to improve dramatically through scale, iteration, and increasing efficiency.
Reduced Labor Costs
Instead of driving a tractor to apply the fertilizer, it integrates into automated irrigation systems.
The upshot is that Nitricity is price competitive even at prototype scale where farms are sunny, far from fertilizer plants, use irrigation, and have alkaline soil. Improvements in cost and efficiency paired with falling solar panel costs threaten to reduce demand for natural gas to make ammonia - somewhere between 5%-10% of global gas demand. Fertilizer is only one example of the possible engineering paradigm shift.
24/7 Process Heat
Natural gas dominates the market for process heat in factories and chemical plants. Renewables under $15/MWh start competing on a direct energy basis with natural gas. The startup Rondo wants to turn cheap, variable electricity into on-demand process heat. The plan is to heat bricks to 2200 F with electric resistance heating. Resistance heaters and bricks are cheap. The facility can remove heat from the bricks as needed. Round trip efficiency can be over 85% - much higher than making hydrogen or synthetic fuels.
Gas is more vulnerable to renewables in applications with regular daily demand than more sporadic use cases like building heat.
Solving Methane Emissions
Many environmental attacks on gas focus on methane leaks. While methane's longevity in the atmosphere is short compared to carbon dioxide, its warming potential per ton is higher. Environmentalists are concerned about leaks from wells, pipelines, and distribution systems.
It turns out that leaks themselves are usually inexpensive to fix, but they are expensive to find. It is hard to know you even have one. A sleepy well might cost as little as $400 in labor per month to operate. Checking for methane leaks requires an expensive tech to haul a $25,000 thermal camera to each remote site. Checking for pipeline leaks requires workers on four-wheelers to trace lines across fields and fences with expensive gas sniffers. Fixing a dump valve or small hole can cost less than $100, but you might spend thousands of dollars to find out you have a leak in the first place.
Low-cost satellites and launch providers change the math. A charity plans to launch MethaneSAT in the fall of 2022 on a SpaceX rocket. The satellite will find point source emissions in better detail than any existing satellite by detecting methane at parts per billion levels with sub-100-meter resolution. The charity estimates the entire project costs $88 million. Data will be public, and the satellite can image most of the globe every few days. There are almost two million oil and gas wells in the US alone. Not to mention the pipelines, landfills, and distribution systems the satellite can cover as well. The cost of leak detection can drop orders of magnitude.
Data allows efficient taxing or repair of leaks. Cities can determine where their gas systems need updating. Satellites move methane emissions from an existential bludgeoning to a straightforward problem.
The Long Game
Exports, tightening supply, carbon pricing, and increasing renewables penetration will push gas more into seasonal and peaking duties over the coming decades. A carbon tax would increase gas consumption by encouraging coal-to-gas switching in the short term. After the coal is gone, it will raise the price in applications where gas has a strong comparative advantage until carbon capture is less costly.
Over time the gas system will become cleaner as methane leaks diminish, new power plants replace the old, synthetic fuel production grows, and DAC options become cheaper than carbon pricing. The politics of carbon pricing will become more palatable once fossil fuels like coal and oil are not setting the everyday marginal price of energy for voters. While drilling might become less common, there will still be a rump of existing wells that produce gas at low prices for decades to come.
The chemical industry uses electricity, gas, and oil based on relative pricing and comparative advantage. Pricier gas, falling electricity prices, transport electrification, and cheap electrochemistry-based technologies could shift the balance towards electricity and oil.
Because new infrastructure is expensive, there is almost certainly going to be some amount of gas generation that remains the cheapest generation even with massive cost declines in other technologies. In 50 years, when our current set of gas power plants starts to reach end-of-life, there might be a surplus of depreciated battery storage that has more value running 20 cycles a year as peak capacity than scrap. Cheap, distributed electricity generation that avoids grid costs is the only technology that could upend this dynamic outside of massive political intervention.
Many climate activists support electrification in anticipation of a cleaner electricity grid. We should begin to anticipate and work towards a better gas system.
NREL Storage Futures Study
FERC Report on Natural Gas Storage
Natural Gas Power Plant Capacity - EIA
EIA Electricity Demand Stats