The Why
The following is part one of a proposal detailing the need for a geothermal focused research organization (FRO). FROs try to push promising technologies through the chasm to reach wide commercialization.
Geothermal's Advantages
The energy industry is going through a long-term deflationary period. Unconventional oil and gas, solar, wind, and batteries have all experienced rapidly falling costs. Every technology comes with tradeoffs. Solar and wind are not dispatchable, batteries have limited duration, and shale hydrocarbons require constant investment and a sophisticated supply chain.
Modern Geothermal provides:
-
High Capacity Factor (24/7 production)
-
Zero Fuel Costs
-
High Power Density (Plants use little land)
-
Provides Electricity, Heat, or Combined Heat and Power
-
Can be Sited Almost Anywhere (Reducing transmission costs)
-
Enough Energy Available to Power the World
Geothermal is a candidate to replace aging nuclear and hydropower plants, given that renewing permits for both types of generators will probably become difficult. It is also great to pair with industrial users that have steady 24/7 electricity demand.
Why don't we have geothermal everywhere? It's too expensive. Geothermal market share growth depends on substantial cost reductions.
Modern Geothermal's Requirements
All that energy is beneath our feet, but it is diffuse and low quality, making cost-effective extraction challenging.
Deep Wells
The Earth's temperature increases as you drill deeper.
Heat engines that convert heat to electricity are more efficient at higher temperatures.
Deep wells produce higher volume and quality of heat, reducing the cost and increasing the effectiveness of generating equipment.
For various reasons, few startups are attempting to drill deep, simple, and cost-effective wells. Their long-term cost goals are above current wholesale electricity prices. The few pursuing a cheaper path have a long technology roadmap or are underfunded. I wrote more about this topic in a blog post.
Widespread Availability
Most existing geothermal power plants drill into hot water (hydrothermal) reservoirs. These resources are rare, making geothermal a niche source of energy. Any attempt to expand the scope of geothermal means drilling without readily available hydrothermal reservoirs and where the thermal gradient is less steep. Not everywhere has volcanoes. Enhanced geothermal systems (EGS), hybrid systems, and closed-loop systems are proposed solutions for hot, dry rock.
Closed-loop systems are like a radiator, only gaining heat through conduction from the rock. Hybrid systems have a closed-loop working fluid but use fracturing techniques to induce convection in reservoir fluid. EGS connects multiple wells through fracturing and circulates fluid between them, using the reservoir rock as extra heat transfer area and volume.
The following proposal is applicable for closed-loop, hybrid systems, and possibly deep EGS with some modifications. More detail on these ideas is available in the same blog post.
No Incentives To Be First
Deep drilling is a high risk, requiring big rewards. Being first isn't rewarded.
Acreage is Not Valuable
Oil and gas resources are highly concentrated. A 100' thick geological formation over several square miles can hold over a billion dollars worth of hydrocarbons. Even if drilling one well is expensive and speculative, the reward of proving valuable acreage can be worth it.
Traditional geothermal wells target rare hydrothermal resources, but the hot, dry rock is everywhere. The point is to make geothermal ubiquitous, after all. Buying a few thousand acres of drilling rights and "proving them up" is not a viable strategy because even near hot spots, those acres are a dime a dozen.
Lack of IP
Attempting to drill wells with off-the-shelf equipment means there is no intellectual property to sell if the drilling techniques are successful. Startups are developing new drilling technology, but they are still years away and have substantial technology risks.
Utility Returns
Geothermal heat has a low value compared to hydrocarbons. Geothermal projects earn utility-like returns instead of lopsided returns that reward risk.
No Reason to Drill the Granite
There are no known valuable resources besides heat deep into the granite. It is rarely thought about by companies that regularly drill.
Drilling Risk Creates a Chasm
Drilling high-risk, technically challenging wells for utility returns does not induce a rush of development capital. If a team proved the basic drilling techniques, portfolios of wells could mitigate individual well risk. Early wells are a public good.
The How
Goals and Vision
Geothermal projects should aim for profitability at $40/MWh to be widely competitive. In 10 years (or less!), projects will need to be profitable at around $20/MWh.
The bare minimum drilling metric that matches the initial goal is reaching 300C rock for $5 million.
If we want cheap, ubiquitous geothermal, then we should practice doing it. Targeting sedimentary basins, geopressured basins, or accepting lower temperatures, like most geothermal startups are doing, has a high probability of being an evolutionary dead end. Science and research only go so far. Cheap drilling allows for more experimentation and science that can unlock further gains. The following ideas ruthlessly focus on simple, cheap wells that are an adaptable platform for complementary technologies.
KISS (Keep it simple, stupid!)
If we want to drill globally at a low cost, simplicity is the only answer. The US is the global drilling technology leader. Drilling costs are higher outside the US for complex technologies like offshore drilling or horizontal, multi-stage fractured wells.
Simplicity can be achieved in the overall design and through embedding complex technology in an easy-to-use technology, like a drill bit. The following proposal focuses on adopting off-the-shelf technologies.
Iterative Approach
Reaching 300C in most of the world requires drilling some of the deepest wells ever drilled. An iterative approach to drilling means starting as simple as possible and making adjustments to develop drilling technology that drills the wells for as simple as possible at the lowest cost possible. More "Let's try a few simple things" than "Let's design a complex solution to this hypothetical problem."
Why It Might Work
A deep, vertical well is less complicated than a conventional oil and gas well or a hydrothermal well. New technology helps.
Traditional Geothermal Drilling Conditions are Horrific
Because of geological activity, rock around hydrothermal reservoirs has massive fractures. Fractures measure in centimeters instead of millimeters. Drilling fluid flows into these fractures instead of circulating out of the hole, causing "lost circulation." Lost circulation often results in getting stuck. In hot drilling conditions, it can lower the pressure of the fluid column, causing drilling fluid to flash to steam.
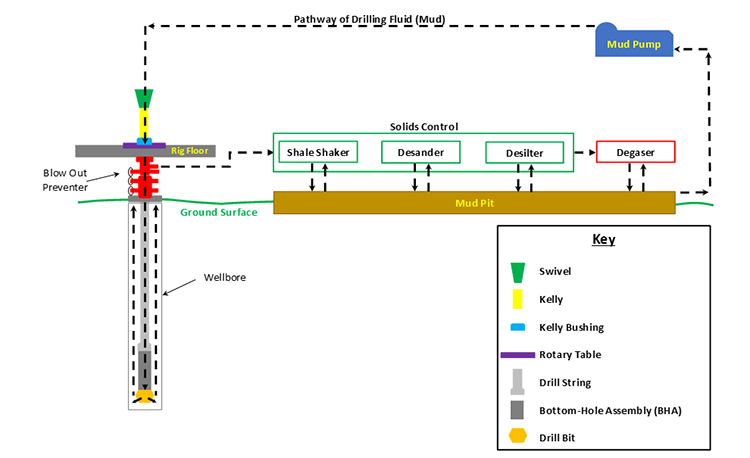
How Drilling Fluid Circulates Source: Greg King © Penn State, licensed CC BY-NC-SA 4.0
Fluid in hydrothermal systems often has hydrogen sulfide (H2S) gas and carbon dioxide (CO2). H2S is incredibly poisonous, and both H2S and CO2 are corrosive, compromising drilling pipes, bits, and other equipment.
Operators run extra casing(pipe) strings to cover up the fractured rock and stop lost circulation. The well has a minimum size needed to move enough hydrothermal fluid to make the project economical. Extra casing strings lead to an explosion in cost as every hole has to be larger (and more expensive). Some geothermal wells have to use titanium casing to handle both temperature and high corrosion instead of cheap carbon steel.
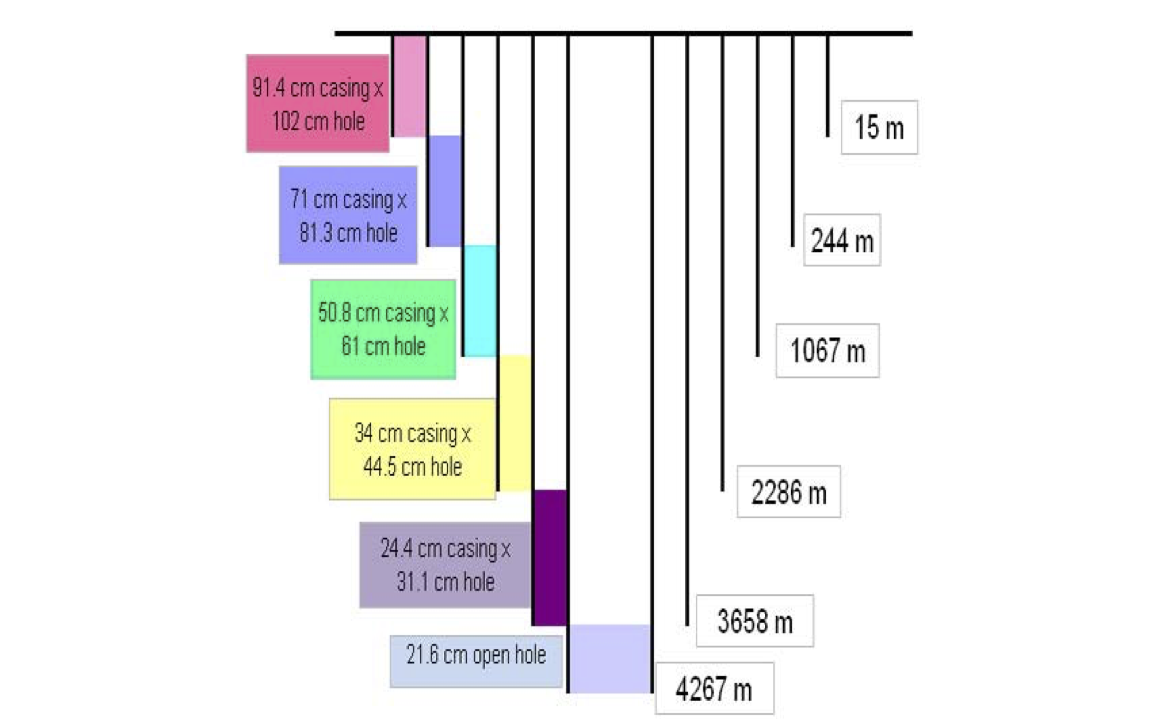
Pipes in pipes in pipes in pipes in pipes. Source: Geothermal Drilling Handbook
In drilling, additional constraints do not add to the cost. They multiply it.
Granite in Benign Geology Simplifies
The deepest wells in the US have all been natural gas wells drilled in the middle of sedimentary basins. Deep basins are often geopressured, meaning the pressure gradient is much steeper than the pressure gradient of water, making drilling difficult.
Geothermal wells can avoid geopressured areas. Additionally, most of the drilling for geothermal will be in igneous rock like granite. Unlike sandstones and shales, granite is solid. It does not cause hole integrity problems.
Away from hydrothermal systems, granite has fewer fractures and is less likely to have H2S and CO2 gas. The University of Utah's FORGE program has drilled two ~10,000' wells into granite without encountering lost circulation or acid gases.
The rock is hard and abrasive, making drilling slow, but drilling in granite removes project requirements, leading to simpler systems.
Better Bits
Until recently, almost all drilling used tri-cone roller bits. These bits have moving parts and seals that limit the hours a bit can drill. In the tough granite, a tri-cone would likely do less than 10'/hr and might not even make 50 hours of drilling. As the bottom hole temperature increases, those hours would decrease further.
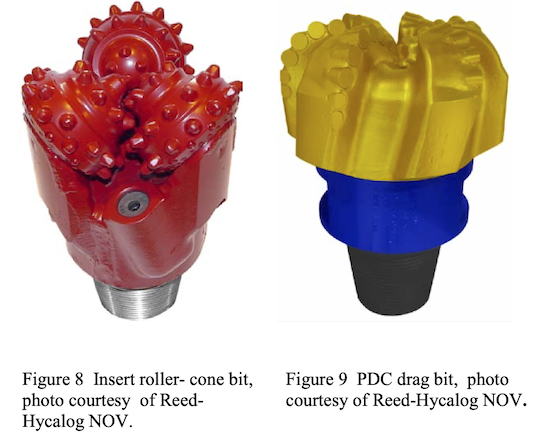
Source: Geothermal Drilling Handbook
Modern designs are polycrystalline diamond compact (PDC) bits. PDCs are much faster. Utah's FORGE wells saw drilling speeds up to 60'/hr in the granite. PDCs have no moving parts or seals and can handle higher temperature drilling conditions much better.
Focus on Vertical Wells
Vertical wells are vastly simpler than directional wells. Once holes start going directional or horizontal, it is harder to get cuttings out. Tools to steer the well are expensive and are sensitive to heat. Drilling is slower in deviated wells as it is harder to transfer weight to the bit. The footage that isn't vertical should be zero or close to it.
Technical Details
Bits and Motors
The vast majority of recent bit technology advances have gone into PDCs. PDCs lose effectiveness or fail when cutters chip or wear. Much of the improvement in drilling speed has come from more durable cutters that allow more aggressive bit designs. Durability is critical for deep geothermal wells because basement rock is hard and abrasive.
PDCs are vulnerable to impact damage. Modern electric rigs have more precise auto drillers (old rigs have an actuator-powered chain that hooks onto the brake handle). Shock subs are available that help keep stretching or compaction in the drill pipe from slamming the bit down onto the rock.
Downhole motors are a requirement for drilling deep holes. Traditionally the drill string is rotated at the surface, turning the bit on the bottom. Drill pipe can twist, making the rotation on bottom zero or even backward in deep wells. PDCs need to rotate consistently. Downhole motors use pressure from the circulating drilling fluid to turn the bit.
Since high-temperature drilling is rare, motor choices are limited. 99%+ of mud motors have elastomers that fail at high temperatures. Turbine motors can handle high temperatures, but they have high RPMs that are too fast for PDCs. The bit designs compatible with turbine motors are old, slow, and expensive.
One choice is to run a conventional mud motor with extra care to circulate fluid regularly to cool it. Bit/motor runs will be shorter as the depth increases, but this is the best option without new motors or modified motors.
Drilling Fluid System
Drilling fluid (or "mud") serves multiple purposes. It carries cuttings out of the hole, provides blowout control with its hydrostatic pressure, cools the bit and motor, and stabilizes the rock exposed from drilling, among other uses.
The mud system can be conventional drilling through the sedimentary rock until the granite. Once in the granite, the simplest solution is pure water with gel sweeps to help hole cleaning.
Basement rock rarely has matrix porosity. Matrix porosity, especially in sandstones, creates a filter cake of drill cuttings on the formation as fluid flows into the rock. The filter cake can stick the bottom hole assembly when tripping out of the hole. Fluid loss additives aren't necessary without matrix porosity. Sedimentary clays can be water sensitive. Operators use additives to prevent clays from absorbing water and expanding or use an oil-based mud as an alternative. Basement rock doesn't react with water, meaning water is acceptable.
Modern rigs have different needs for hole cleaning than traditional rigs that drilled deep vertical holes. Mud pumps have gotten much larger, and PDC bits create smaller diameter cuttings than tri-cone bits. As a result, higher velocity mud can carry the smaller cuttings without adding gel or polymer to increase viscosity. The slower drilling speed of basement rock also helps. Gel sweeps every so often and before trips provide hole cleaning insurance.
Not only is a simple mud system cheap, but there are fewer additives that need to be high temperature rated. Mud weights (density) usually range from 9.2-10 lbs/gal in non-geopressured reservoirs. A straight water system will be around 8.5 lbs/gal, reducing lost circulation risk.
Heavier drilling fluids compress rock more, slowing down drilling penetration rate and increasing bit wear. Lighter fluids like straight water drill better.
Using water also reduces friction loss, lowering circulating pressure.
Modern rigs usually use closed pits. Fluid from the hole goes over a "shale shaker" that removes cuttings and flows into tanks for reuse. The high bottom-hole temperature will require a mud cooler before the shale shaker to take the heat out of the system and prevent worker injury. Closed pit systems sometimes need a centrifuge to remove small particles from the mud.
It might be possible in the future to use old-school "open drilling pits." Water could be routed straight to what looks like a small pond. The settling time in the pit is long, so solids fall out, and the mud cools. Rentals like the mud cooler and the centrifuge are no longer needed. There is no need to buy shale shaker screens. The downside is that it is more difficult to tell if fluid is being gained or lost downhole, increasing safety risk, making it inadvisable for early wells with high uncertainty.
Drilling Rig
Horizontal wells require big drilling rigs. There are rigs capable of drilling to 10 km (~32,000') in the US lower 48. There are few rigs designed to go deeper.
Drilling rigs are complex systems, meaning that more than one component limits the depth. For that reason, ~32,000' is the logical limit to drilling depth until there is consistent demand for deeper drilling.
Most rigs in this class are electric, providing an operating cost advantage. Deep wells take long enough to drill that an electric rig can use utility power instead of running a diesel generator. The location is quieter and cleaner besides having lower fuel costs.
Example of a 10 km rig.
Safety
Drilling accidents can cost orders of magnitude more than projected drilling costs. Most operators buy blowout insurance for wells, but insurers may balk at quoting a one-of-a-kind super deep vertical well. Minimizing blowout and safety risks has to be a priority.
The good news is that the risks are lower than hydrothermal wells or deep basin gas wells.
High-pressure gas is always a concern. Gas lightens the fluid column, allowing more reservoir fluid to flow into the well. Gas might be flammable hydrocarbons or can carry dangerous components like H2S.
Gas in the basement rock is relatively rare. If present, it typically migrates to the top of the basement, where it will be easier to handle. Even if there is gas after the transition to the basement drilling fluid program, there will be plenty of casing in place to help manage a kick. Bullhead pumping fluid into a reservoir could damage its oil or hydrothermal production. A closed-loop geothermal well does not need productivity, so bull heading can be used, quickly controlling the well. Unlike deep basin drilling, the pressure gradient is typically low.
When drilling in areas with extreme temperature gradients, steam can be present in reservoirs. If not handled carefully, it can blow out and might contain H2S from a hydrothermal system. High bottom-hole pressures keep water in the liquid phase where the temperature gradient is moderate. H2S forms by the interaction of water with rock in cycling hydrothermal systems. Typical areas are less likely to have H2S gas.
Loss of circulation is another risk geothermal wells face. Lost circulation reduces the height of the fluid column, which can flash to steam. Luckily this problem is more severe in geologically active areas. A moderate temperature gradient in more normal geological areas means more "insurance" pressure on the fluid. The column cools more as it takes longer to circulate from the hole for a given bottom hole temperature. The depths at which temperatures become high enough to cause steam flashing have less of the fractures that cause loss circulation in the first place.
Even with the more favorable risk profile, extra training, double-checking blowout preventers (BOPs), and the temperature rating of their rubbers, maintaining H2S readiness and other precautions are necessary to keep risk to the minimum. No drilling is ever risk-free.
Casing and Completions
Steel pipe called casing keeps the well from collapsing. Cement is pumped around the outside of the casing to isolate productive rock formations that produce hydrocarbons or hydrothermal fluid and protect the casing from corrosive rock formations. Setting casing and cementing make up over 40% of the cost in hydrothermal wells. Reducing casing requirements are one of the best opportunities to reduce costs.
As is typical, this design uses a surface casing string to protect groundwater. The well design calls for drilling and casing the sedimentary portion of the well conventionally. If the basement is shallow, <8000', it may be necessary to the casing string deeper in the basement to cover lost circulation zones or handle kicks. The casing design may not meet the strictest requirements for kick tolerance. Requiring ultra-conservative standards almost ensure that geothermal will never be significant with conventional technology.
The basement allows us to delete other casing strings.
On the first well, the string to delete will be the production string. Since there is no productive zone to isolate and granite does not cave in, it is unnecessary. The possible complications are cuttings falling out of fractures or corrosion/scaling from reservoir fluid. If these problems surface, it may be possible to remedy them with a sealant rather than casing and cement. The sealant strategy can also reduce the tubing requirement because fluid can travel down the bare rock annulus. The geothermal startup, Eavor, uses this strategy in its open hole wells, albeit at lower temperatures. A plan that adds gunk while drilling can also decrease the chances of losing fluid to fractures and improve safety.
Why isn't gunk feasible in oil or hydrothermal wells? It impairs the productivity of reservoirs. Granite is solid rock with only fractures. Sedimentary rocks have matrix porosity and are softer, making gunk infeasible. The value of gunk is highest in the shallower portions of the well. Even if gunk is temperature limited, it may not matter.
Less casing and smaller holes lead to drastic cost reductions.
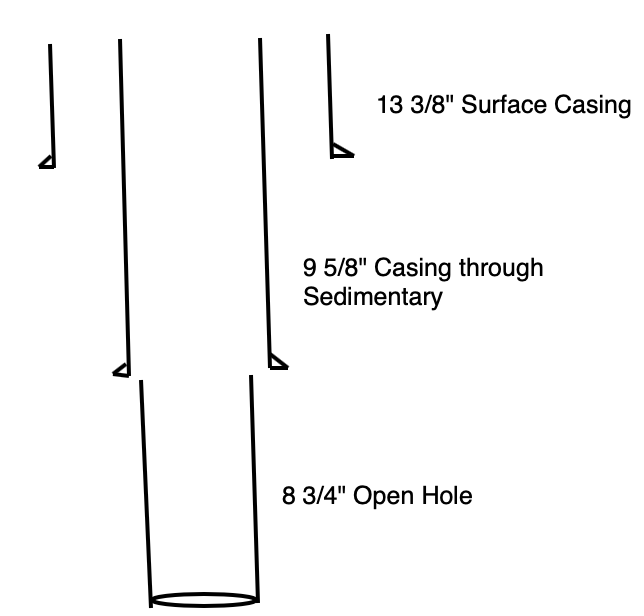
Utah FORGE Real-World Validation
Drilling needs to be around 20'/hr, and bottom hole assemblies need to last for 1000'-2000' of drilling granite to achieve the cost targets. Utah FORGE has released detailed daily drilling reports and analyses for several of their recent wells. Let's look at the drilling runs in the granite:
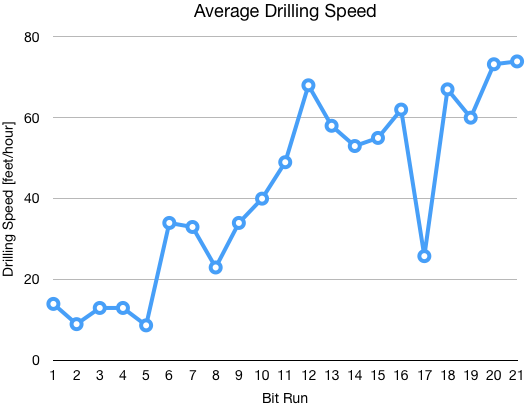
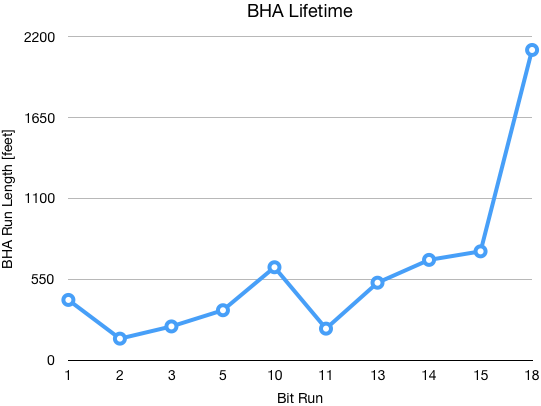
Data from University of Utah FORGE Project
The BHA durability chart only includes runs that ended because of bit wear. Once bits become more durable, runs are more likely to end because of casing points or other activities. As is typical with drilling in a new area, early bit runs are suboptimal. Improvement comes through testing different designs informed by the performance of previous bits.
FORGE has a wide range of objectives. They are studying rock properties, trying to drill slant wells, etc. Their work has proven the capabilities of modern bits in granite. The drilling has also shown that there are regions with favorable temperature gradients with benign drilling conditions.
Further Testing
Future Cost Improvements
-
High-Temperature Motors
There are two main development paths for high-temperature motors. The optimal choice is durable, long-life turbine motors geared for PDC RPMs or another type of elastomer-free design. The crutch choice is to add another section to a high RPM turbine motor that gears down the bit RPMs.
Baker Hughes developed a traditional mud motor with no elastomers for drilling a hot well in Iceland, but it is not regularly available.
These motors are the most critical upgrade. They reduce trips by increasing BHA longevity in the deepest portions of the wellbore, where changing a bottom hole assembly costs the most. Even if an individual motor costs $40,000, it would be worth it if it prevented one trip.
-
Optimized Bits and Cutters
As we saw with Utah FORGE, operators and vendors optimize bits through trial and error. The bigger the market, the more R+D resources are devoted to it. Eventually, improvements come to most expensive processes like cutter design.
-
Ultra-Deep Drilling Rigs
Use lessons from drilling to design rigs capable of 40,000'-50,000' depths. New rigs need bigger pumps, drill pipe, pipe capacity, top drives, and derricks. Rig companies will build custom rigs with an operator signing a 2-4 year drilling contract.
-
Further Simplification
I only have so much creativity. Iteration will discover better ways. Especially when the sedimentary portion and tubing costs quickly dominate costs.
Simplistic Cost Model
There is a fixed cost for mobilizing a rig, creating a site, and setting the surface casing to protect water resources.
- $300,000 for rig mobilization, permitting, surface payments, and location construction.
Then there is a typical cost per foot for the sedimentary drilling.
- $100/ft for drilling, casing, and cementing sedimentary rock 100' into granite basement.
If the sedimentary rock extends greater than 10,000', then this is an underestimate. Operators should avoid deep sedimentary basins, anyway.
Next is the uncased granite drilling portion. Given that almost all future cost decreases come from this section, the formula allows variation for drilling speed and BHA durability.
The calculations are in the appendix below. Costs are conservative because they roll many smaller associated services into them. The biggest factor in early costs is increasing BHA lifetime. Drilling speed plays a larger role until sedimentary and tubing costs dominate.
Well Attributes:
-
32,000' depth with 8000' of sedimentary rock. The model assumes sealed bare rock as casing and large diameter pipe as tubing.
-
Cost using best FORGE runs: $2.98 Million.
-
Aspirational Cost with faster and more durable BHAs (not a limit): $2.31 Million.
-
Aspirational 50,000' well: $3.12 Million.
The costs might seem unimaginably low, but that is the point of drilling a hole with the absolute simplest possible drilling configuration. Better bits drive the granite drilling cost per foot even lower. Over the last decade, massive improvements in drilling productivity have been the norm. According to EIA, a drilling rig in most regions is 10x more productive. A combination of optimizing for granite drilling and continued fundamental improvements in motors and PDC cutters mean wild long-term cost decreases are possible.
The model includes tubing for a closed-loop system but not any completion activity for hybrid or EGS systems. US drilling prices form the basis for this model. Other markets will require some level of service localization to reach these costs. Germany is the most notable international market. It has a 220 euro/MWh feed-in tariff for geothermal electricity. Geothermal projects selling into US wholesale electricity market would be lucky to earn $40/MWh.
PDC bits are dumb pieces of mass with genius designs. Motors have few moving parts. Gravity and water pressure power the drilling system. If diamond cutters keep improving, conventional drilling will beat trying to put fancy, new drilling technologies in the hole for a long time.
Next Steps
Drilling could commence as fast as 90 days in a state with drilling-friendly regulations and a location with privately-owned minerals. Testing high-temperature motors and gunk is valuable enough to try on the first well. An estimate of 180 days might be more realistic to start drilling.
Each rig and program has its learning curve dealing with surprises. The first well will be more than $3 million, especially if the high-temperature motor tests fail. $10 million might be more realistic to cover problems and completion testing for hybrid or EGS systems.
Outlook
I would guess that the geothermal industry's status quo development has a 5% chance of developing technology that can earn more than 10% market share of electricity generation. Trying to drill deep, vertical wells with off-the-shelf technology is an attempt to improve those odds and spur the development of needed complementary technologies.
What do we do with this possible drilling cost plunge? Cheap vertical wells can't drive double-digit market share alone, but it is the first step. Part two will cover the heat transfer area/volume problem. Part three will cover the heat engine problem.
-
Geothermal Drilling Handbook
-
10 km Temperature Map
-
Basement Depth Map
-
FORGE Drilling Data and Reports
Drilling Cost Model
Written in Python. Below numbers are filled in for the aggressive case.
import math
#Cost Factors
fixed_cost = 300000
sedimentary_cost_per_foot = 100
wellbore_count = 1
drilling_feet_per_hour = 100
non_trip_utilization = 0.9
BHA_lifetime_feet = 10000
drilling_day_rate = 40000
BHA_cost = 25000
tubing_cost_per_foot = 20
#Well Settings
sedimentary_footage = 8000
total_depth_feet = 50000
granite_interval_feet = total_depth_feet - sedimentary_footage
tripping_speed_feet_per_hour = 2000
#Calculation
cost_to_granite = fixed_cost*wellbore_count + sedimentary_cost_per_foot*sedimentary_footage*wellbore_count
granite_drilling_hours = granite_interval_feet/(drilling_feet_per_hour*non_trip_utilization)
number_of_trips = math.ceil(granite_interval_feet/BHA_lifetime_feet)
average_granite_trip_distance = sedimentary_footage + granite_interval_feet/2
granite_trip_hours = (average_granite_trip_distance * number_of_trips) / tripping_speed_feet_per_hour
total_rig_hours = granite_trip_hours + granite_drilling_hours
total_dayrate_costs = (granite_trip_hours + granite_drilling_hours)/24 * drilling_day_rate
total_BHA_costs = BHA_cost * number_of_trips
tubing_cost = total_depth_feet * tubing_cost_per_foot
granite_cost = total_dayrate_costs + total_BHA_costs
total_cost = cost_to_granite + granite_cost + tubing_cost
print("Number of trips: ", number_of_trips)
print("Total Drilling Cost: $",round(total_cost/1000000,2), " Million")
print("Granite $/ft: ",round(granite_cost/granite_interval_feet,2))
Geothermal's Path to Relevance: Cheap Drilling
2021 October 24 Twitter Substack See all postsThe first in a three part series detailing technology needed to make geothermal electricity economical. Thanks to James, Michael, Martin, and Matt for technical help. Any mistakes are my own.
The Why
The following is part one of a proposal detailing the need for a geothermal focused research organization (FRO). FROs try to push promising technologies through the chasm to reach wide commercialization.
Geothermal's Advantages
The energy industry is going through a long-term deflationary period. Unconventional oil and gas, solar, wind, and batteries have all experienced rapidly falling costs. Every technology comes with tradeoffs. Solar and wind are not dispatchable, batteries have limited duration, and shale hydrocarbons require constant investment and a sophisticated supply chain.
Modern Geothermal provides:
High Capacity Factor (24/7 production)
Zero Fuel Costs
High Power Density (Plants use little land)
Provides Electricity, Heat, or Combined Heat and Power
Can be Sited Almost Anywhere (Reducing transmission costs)
Enough Energy Available to Power the World
Geothermal is a candidate to replace aging nuclear and hydropower plants, given that renewing permits for both types of generators will probably become difficult. It is also great to pair with industrial users that have steady 24/7 electricity demand.
Why don't we have geothermal everywhere? It's too expensive. Geothermal market share growth depends on substantial cost reductions.
Modern Geothermal's Requirements
All that energy is beneath our feet, but it is diffuse and low quality, making cost-effective extraction challenging.
Deep Wells
The Earth's temperature increases as you drill deeper.
Heat engines that convert heat to electricity are more efficient at higher temperatures.
Deep wells produce higher volume and quality of heat, reducing the cost and increasing the effectiveness of generating equipment.
For various reasons, few startups are attempting to drill deep, simple, and cost-effective wells. Their long-term cost goals are above current wholesale electricity prices. The few pursuing a cheaper path have a long technology roadmap or are underfunded. I wrote more about this topic in a blog post.
Widespread Availability
Most existing geothermal power plants drill into hot water (hydrothermal) reservoirs. These resources are rare, making geothermal a niche source of energy. Any attempt to expand the scope of geothermal means drilling without readily available hydrothermal reservoirs and where the thermal gradient is less steep. Not everywhere has volcanoes. Enhanced geothermal systems (EGS), hybrid systems, and closed-loop systems are proposed solutions for hot, dry rock.
Closed-loop systems are like a radiator, only gaining heat through conduction from the rock. Hybrid systems have a closed-loop working fluid but use fracturing techniques to induce convection in reservoir fluid. EGS connects multiple wells through fracturing and circulates fluid between them, using the reservoir rock as extra heat transfer area and volume.
The following proposal is applicable for closed-loop, hybrid systems, and possibly deep EGS with some modifications. More detail on these ideas is available in the same blog post.
No Incentives To Be First
Deep drilling is a high risk, requiring big rewards. Being first isn't rewarded.
Acreage is Not Valuable
Oil and gas resources are highly concentrated. A 100' thick geological formation over several square miles can hold over a billion dollars worth of hydrocarbons. Even if drilling one well is expensive and speculative, the reward of proving valuable acreage can be worth it.
Traditional geothermal wells target rare hydrothermal resources, but the hot, dry rock is everywhere. The point is to make geothermal ubiquitous, after all. Buying a few thousand acres of drilling rights and "proving them up" is not a viable strategy because even near hot spots, those acres are a dime a dozen.
Lack of IP
Attempting to drill wells with off-the-shelf equipment means there is no intellectual property to sell if the drilling techniques are successful. Startups are developing new drilling technology, but they are still years away and have substantial technology risks.
Utility Returns
Geothermal heat has a low value compared to hydrocarbons. Geothermal projects earn utility-like returns instead of lopsided returns that reward risk.
No Reason to Drill the Granite
There are no known valuable resources besides heat deep into the granite. It is rarely thought about by companies that regularly drill.
Drilling Risk Creates a Chasm
Drilling high-risk, technically challenging wells for utility returns does not induce a rush of development capital. If a team proved the basic drilling techniques, portfolios of wells could mitigate individual well risk. Early wells are a public good.
The How
Goals and Vision
Geothermal projects should aim for profitability at $40/MWh to be widely competitive. In 10 years (or less!), projects will need to be profitable at around $20/MWh.
The bare minimum drilling metric that matches the initial goal is reaching 300C rock for $5 million.
If we want cheap, ubiquitous geothermal, then we should practice doing it. Targeting sedimentary basins, geopressured basins, or accepting lower temperatures, like most geothermal startups are doing, has a high probability of being an evolutionary dead end. Science and research only go so far. Cheap drilling allows for more experimentation and science that can unlock further gains. The following ideas ruthlessly focus on simple, cheap wells that are an adaptable platform for complementary technologies.
KISS (Keep it simple, stupid!)
If we want to drill globally at a low cost, simplicity is the only answer. The US is the global drilling technology leader. Drilling costs are higher outside the US for complex technologies like offshore drilling or horizontal, multi-stage fractured wells.
Simplicity can be achieved in the overall design and through embedding complex technology in an easy-to-use technology, like a drill bit. The following proposal focuses on adopting off-the-shelf technologies.
Iterative Approach
Reaching 300C in most of the world requires drilling some of the deepest wells ever drilled. An iterative approach to drilling means starting as simple as possible and making adjustments to develop drilling technology that drills the wells for as simple as possible at the lowest cost possible. More "Let's try a few simple things" than "Let's design a complex solution to this hypothetical problem."
Why It Might Work
A deep, vertical well is less complicated than a conventional oil and gas well or a hydrothermal well. New technology helps.
Traditional Geothermal Drilling Conditions are Horrific
Because of geological activity, rock around hydrothermal reservoirs has massive fractures. Fractures measure in centimeters instead of millimeters. Drilling fluid flows into these fractures instead of circulating out of the hole, causing "lost circulation." Lost circulation often results in getting stuck. In hot drilling conditions, it can lower the pressure of the fluid column, causing drilling fluid to flash to steam.
How Drilling Fluid Circulates Source: Greg King © Penn State, licensed CC BY-NC-SA 4.0
Fluid in hydrothermal systems often has hydrogen sulfide (H2S) gas and carbon dioxide (CO2). H2S is incredibly poisonous, and both H2S and CO2 are corrosive, compromising drilling pipes, bits, and other equipment.
Operators run extra casing(pipe) strings to cover up the fractured rock and stop lost circulation. The well has a minimum size needed to move enough hydrothermal fluid to make the project economical. Extra casing strings lead to an explosion in cost as every hole has to be larger (and more expensive). Some geothermal wells have to use titanium casing to handle both temperature and high corrosion instead of cheap carbon steel.
Pipes in pipes in pipes in pipes in pipes. Source: Geothermal Drilling Handbook
In drilling, additional constraints do not add to the cost. They multiply it.
Granite in Benign Geology Simplifies
The deepest wells in the US have all been natural gas wells drilled in the middle of sedimentary basins. Deep basins are often geopressured, meaning the pressure gradient is much steeper than the pressure gradient of water, making drilling difficult.
Geothermal wells can avoid geopressured areas. Additionally, most of the drilling for geothermal will be in igneous rock like granite. Unlike sandstones and shales, granite is solid. It does not cause hole integrity problems.
Away from hydrothermal systems, granite has fewer fractures and is less likely to have H2S and CO2 gas. The University of Utah's FORGE program has drilled two ~10,000' wells into granite without encountering lost circulation or acid gases.
The rock is hard and abrasive, making drilling slow, but drilling in granite removes project requirements, leading to simpler systems.
Better Bits
Until recently, almost all drilling used tri-cone roller bits. These bits have moving parts and seals that limit the hours a bit can drill. In the tough granite, a tri-cone would likely do less than 10'/hr and might not even make 50 hours of drilling. As the bottom hole temperature increases, those hours would decrease further.
Source: Geothermal Drilling Handbook
Modern designs are polycrystalline diamond compact (PDC) bits. PDCs are much faster. Utah's FORGE wells saw drilling speeds up to 60'/hr in the granite. PDCs have no moving parts or seals and can handle higher temperature drilling conditions much better.
Focus on Vertical Wells
Vertical wells are vastly simpler than directional wells. Once holes start going directional or horizontal, it is harder to get cuttings out. Tools to steer the well are expensive and are sensitive to heat. Drilling is slower in deviated wells as it is harder to transfer weight to the bit. The footage that isn't vertical should be zero or close to it.
Technical Details
Bits and Motors
The vast majority of recent bit technology advances have gone into PDCs. PDCs lose effectiveness or fail when cutters chip or wear. Much of the improvement in drilling speed has come from more durable cutters that allow more aggressive bit designs. Durability is critical for deep geothermal wells because basement rock is hard and abrasive.
PDCs are vulnerable to impact damage. Modern electric rigs have more precise auto drillers (old rigs have an actuator-powered chain that hooks onto the brake handle). Shock subs are available that help keep stretching or compaction in the drill pipe from slamming the bit down onto the rock.
Downhole motors are a requirement for drilling deep holes. Traditionally the drill string is rotated at the surface, turning the bit on the bottom. Drill pipe can twist, making the rotation on bottom zero or even backward in deep wells. PDCs need to rotate consistently. Downhole motors use pressure from the circulating drilling fluid to turn the bit.
Since high-temperature drilling is rare, motor choices are limited. 99%+ of mud motors have elastomers that fail at high temperatures. Turbine motors can handle high temperatures, but they have high RPMs that are too fast for PDCs. The bit designs compatible with turbine motors are old, slow, and expensive.
One choice is to run a conventional mud motor with extra care to circulate fluid regularly to cool it. Bit/motor runs will be shorter as the depth increases, but this is the best option without new motors or modified motors.
Drilling Fluid System
Drilling fluid (or "mud") serves multiple purposes. It carries cuttings out of the hole, provides blowout control with its hydrostatic pressure, cools the bit and motor, and stabilizes the rock exposed from drilling, among other uses.
The mud system can be conventional drilling through the sedimentary rock until the granite. Once in the granite, the simplest solution is pure water with gel sweeps to help hole cleaning.
Basement rock rarely has matrix porosity. Matrix porosity, especially in sandstones, creates a filter cake of drill cuttings on the formation as fluid flows into the rock. The filter cake can stick the bottom hole assembly when tripping out of the hole. Fluid loss additives aren't necessary without matrix porosity. Sedimentary clays can be water sensitive. Operators use additives to prevent clays from absorbing water and expanding or use an oil-based mud as an alternative. Basement rock doesn't react with water, meaning water is acceptable.
Modern rigs have different needs for hole cleaning than traditional rigs that drilled deep vertical holes. Mud pumps have gotten much larger, and PDC bits create smaller diameter cuttings than tri-cone bits. As a result, higher velocity mud can carry the smaller cuttings without adding gel or polymer to increase viscosity. The slower drilling speed of basement rock also helps. Gel sweeps every so often and before trips provide hole cleaning insurance.
Not only is a simple mud system cheap, but there are fewer additives that need to be high temperature rated. Mud weights (density) usually range from 9.2-10 lbs/gal in non-geopressured reservoirs. A straight water system will be around 8.5 lbs/gal, reducing lost circulation risk.
Heavier drilling fluids compress rock more, slowing down drilling penetration rate and increasing bit wear. Lighter fluids like straight water drill better.
Using water also reduces friction loss, lowering circulating pressure.
Modern rigs usually use closed pits. Fluid from the hole goes over a "shale shaker" that removes cuttings and flows into tanks for reuse. The high bottom-hole temperature will require a mud cooler before the shale shaker to take the heat out of the system and prevent worker injury. Closed pit systems sometimes need a centrifuge to remove small particles from the mud.
It might be possible in the future to use old-school "open drilling pits." Water could be routed straight to what looks like a small pond. The settling time in the pit is long, so solids fall out, and the mud cools. Rentals like the mud cooler and the centrifuge are no longer needed. There is no need to buy shale shaker screens. The downside is that it is more difficult to tell if fluid is being gained or lost downhole, increasing safety risk, making it inadvisable for early wells with high uncertainty.
Drilling Rig
Horizontal wells require big drilling rigs. There are rigs capable of drilling to 10 km (~32,000') in the US lower 48. There are few rigs designed to go deeper.
Drilling rigs are complex systems, meaning that more than one component limits the depth. For that reason, ~32,000' is the logical limit to drilling depth until there is consistent demand for deeper drilling.
Most rigs in this class are electric, providing an operating cost advantage. Deep wells take long enough to drill that an electric rig can use utility power instead of running a diesel generator. The location is quieter and cleaner besides having lower fuel costs.
Example of a 10 km rig.
Safety
Drilling accidents can cost orders of magnitude more than projected drilling costs. Most operators buy blowout insurance for wells, but insurers may balk at quoting a one-of-a-kind super deep vertical well. Minimizing blowout and safety risks has to be a priority.
The good news is that the risks are lower than hydrothermal wells or deep basin gas wells.
High-pressure gas is always a concern. Gas lightens the fluid column, allowing more reservoir fluid to flow into the well. Gas might be flammable hydrocarbons or can carry dangerous components like H2S.
Gas in the basement rock is relatively rare. If present, it typically migrates to the top of the basement, where it will be easier to handle. Even if there is gas after the transition to the basement drilling fluid program, there will be plenty of casing in place to help manage a kick. Bullhead pumping fluid into a reservoir could damage its oil or hydrothermal production. A closed-loop geothermal well does not need productivity, so bull heading can be used, quickly controlling the well. Unlike deep basin drilling, the pressure gradient is typically low.
When drilling in areas with extreme temperature gradients, steam can be present in reservoirs. If not handled carefully, it can blow out and might contain H2S from a hydrothermal system. High bottom-hole pressures keep water in the liquid phase where the temperature gradient is moderate. H2S forms by the interaction of water with rock in cycling hydrothermal systems. Typical areas are less likely to have H2S gas.
Loss of circulation is another risk geothermal wells face. Lost circulation reduces the height of the fluid column, which can flash to steam. Luckily this problem is more severe in geologically active areas. A moderate temperature gradient in more normal geological areas means more "insurance" pressure on the fluid. The column cools more as it takes longer to circulate from the hole for a given bottom hole temperature. The depths at which temperatures become high enough to cause steam flashing have less of the fractures that cause loss circulation in the first place.
Even with the more favorable risk profile, extra training, double-checking blowout preventers (BOPs), and the temperature rating of their rubbers, maintaining H2S readiness and other precautions are necessary to keep risk to the minimum. No drilling is ever risk-free.
Casing and Completions
Steel pipe called casing keeps the well from collapsing. Cement is pumped around the outside of the casing to isolate productive rock formations that produce hydrocarbons or hydrothermal fluid and protect the casing from corrosive rock formations. Setting casing and cementing make up over 40% of the cost in hydrothermal wells. Reducing casing requirements are one of the best opportunities to reduce costs.
As is typical, this design uses a surface casing string to protect groundwater. The well design calls for drilling and casing the sedimentary portion of the well conventionally. If the basement is shallow, <8000', it may be necessary to the casing string deeper in the basement to cover lost circulation zones or handle kicks. The casing design may not meet the strictest requirements for kick tolerance. Requiring ultra-conservative standards almost ensure that geothermal will never be significant with conventional technology.
The basement allows us to delete other casing strings.
On the first well, the string to delete will be the production string. Since there is no productive zone to isolate and granite does not cave in, it is unnecessary. The possible complications are cuttings falling out of fractures or corrosion/scaling from reservoir fluid. If these problems surface, it may be possible to remedy them with a sealant rather than casing and cement. The sealant strategy can also reduce the tubing requirement because fluid can travel down the bare rock annulus. The geothermal startup, Eavor, uses this strategy in its open hole wells, albeit at lower temperatures. A plan that adds gunk while drilling can also decrease the chances of losing fluid to fractures and improve safety.
Why isn't gunk feasible in oil or hydrothermal wells? It impairs the productivity of reservoirs. Granite is solid rock with only fractures. Sedimentary rocks have matrix porosity and are softer, making gunk infeasible. The value of gunk is highest in the shallower portions of the well. Even if gunk is temperature limited, it may not matter.
Less casing and smaller holes lead to drastic cost reductions.
Utah FORGE Real-World Validation
Drilling needs to be around 20'/hr, and bottom hole assemblies need to last for 1000'-2000' of drilling granite to achieve the cost targets. Utah FORGE has released detailed daily drilling reports and analyses for several of their recent wells. Let's look at the drilling runs in the granite:
Data from University of Utah FORGE Project
The BHA durability chart only includes runs that ended because of bit wear. Once bits become more durable, runs are more likely to end because of casing points or other activities. As is typical with drilling in a new area, early bit runs are suboptimal. Improvement comes through testing different designs informed by the performance of previous bits.
FORGE has a wide range of objectives. They are studying rock properties, trying to drill slant wells, etc. Their work has proven the capabilities of modern bits in granite. The drilling has also shown that there are regions with favorable temperature gradients with benign drilling conditions.
Further Testing
Future Cost Improvements
High-Temperature Motors
There are two main development paths for high-temperature motors. The optimal choice is durable, long-life turbine motors geared for PDC RPMs or another type of elastomer-free design. The crutch choice is to add another section to a high RPM turbine motor that gears down the bit RPMs.
Baker Hughes developed a traditional mud motor with no elastomers for drilling a hot well in Iceland, but it is not regularly available.
These motors are the most critical upgrade. They reduce trips by increasing BHA longevity in the deepest portions of the wellbore, where changing a bottom hole assembly costs the most. Even if an individual motor costs $40,000, it would be worth it if it prevented one trip.
Optimized Bits and Cutters
As we saw with Utah FORGE, operators and vendors optimize bits through trial and error. The bigger the market, the more R+D resources are devoted to it. Eventually, improvements come to most expensive processes like cutter design.
Ultra-Deep Drilling Rigs
Use lessons from drilling to design rigs capable of 40,000'-50,000' depths. New rigs need bigger pumps, drill pipe, pipe capacity, top drives, and derricks. Rig companies will build custom rigs with an operator signing a 2-4 year drilling contract.
Further Simplification
I only have so much creativity. Iteration will discover better ways. Especially when the sedimentary portion and tubing costs quickly dominate costs.
Simplistic Cost Model
There is a fixed cost for mobilizing a rig, creating a site, and setting the surface casing to protect water resources.
Then there is a typical cost per foot for the sedimentary drilling.
If the sedimentary rock extends greater than 10,000', then this is an underestimate. Operators should avoid deep sedimentary basins, anyway.
Next is the uncased granite drilling portion. Given that almost all future cost decreases come from this section, the formula allows variation for drilling speed and BHA durability.
The calculations are in the appendix below. Costs are conservative because they roll many smaller associated services into them. The biggest factor in early costs is increasing BHA lifetime. Drilling speed plays a larger role until sedimentary and tubing costs dominate.
Well Attributes:
32,000' depth with 8000' of sedimentary rock. The model assumes sealed bare rock as casing and large diameter pipe as tubing.
Cost using best FORGE runs: $2.98 Million.
Aspirational Cost with faster and more durable BHAs (not a limit): $2.31 Million.
Aspirational 50,000' well: $3.12 Million.
The costs might seem unimaginably low, but that is the point of drilling a hole with the absolute simplest possible drilling configuration. Better bits drive the granite drilling cost per foot even lower. Over the last decade, massive improvements in drilling productivity have been the norm. According to EIA, a drilling rig in most regions is 10x more productive. A combination of optimizing for granite drilling and continued fundamental improvements in motors and PDC cutters mean wild long-term cost decreases are possible.
The model includes tubing for a closed-loop system but not any completion activity for hybrid or EGS systems. US drilling prices form the basis for this model. Other markets will require some level of service localization to reach these costs. Germany is the most notable international market. It has a 220 euro/MWh feed-in tariff for geothermal electricity. Geothermal projects selling into US wholesale electricity market would be lucky to earn $40/MWh.
PDC bits are dumb pieces of mass with genius designs. Motors have few moving parts. Gravity and water pressure power the drilling system. If diamond cutters keep improving, conventional drilling will beat trying to put fancy, new drilling technologies in the hole for a long time.
Next Steps
Drilling could commence as fast as 90 days in a state with drilling-friendly regulations and a location with privately-owned minerals. Testing high-temperature motors and gunk is valuable enough to try on the first well. An estimate of 180 days might be more realistic to start drilling.
Each rig and program has its learning curve dealing with surprises. The first well will be more than $3 million, especially if the high-temperature motor tests fail. $10 million might be more realistic to cover problems and completion testing for hybrid or EGS systems.
Outlook
I would guess that the geothermal industry's status quo development has a 5% chance of developing technology that can earn more than 10% market share of electricity generation. Trying to drill deep, vertical wells with off-the-shelf technology is an attempt to improve those odds and spur the development of needed complementary technologies.
What do we do with this possible drilling cost plunge? Cheap vertical wells can't drive double-digit market share alone, but it is the first step. Part two will cover the heat transfer area/volume problem. Part three will cover the heat engine problem.
Geothermal Drilling Handbook
10 km Temperature Map
Basement Depth Map
FORGE Drilling Data and Reports
Drilling Cost Model
Written in Python. Below numbers are filled in for the aggressive case.